Frank J. Dainello and Roland Roberts
Sustainable vegetable farming is a relatively high risk, high cost per acre business requiring intensive management. Successful vegetable growers manage capital, and marketing competently. Growers design and implement systems of culture which include crop and variety selection, crop rotation, soil fertilization, land selection, tillage, integrated pest management (insect, disease and weed control), transplant production and/or use, seedbed preparation, seeding, irrigation, windbreak management, pollination (bee management), harvesting, handling and packaging and sales. Vegetable production differs from other crop production enterprises. These crops are perishable in nature, must be free from blemishes, and, have narrow market windows. Consequently, cultural operations must be accomplished in a more precise and timely manner to deliver high quality products to markets on schedule.
Grower profit and loss are affected by uncontrollable factors including weather, market fluctuations, pest infestations, and legislation. Therefore, growers must pay close attention to variables which they can control. Timely implementation of effective cultural practices enables careful growers to minimize or totally avoid pitfalls. Wise selection of cultural practices improves production efficiency, lowers production cost and increases profitability. As with most successful businesses, careful planning greatly enhances the incidences of success.
The Commercial Vegetable Production Guide, published by AgriLife Extension is a useful reference which outlines specific inputs and optimal growing conditions required to produce over 30 vegetable crops. The guide is revised biennially in order to provide the latest production information to producers. (Oder form for this guide can be found at the back of this publication).
Crop Selection
Selection of vegetable kinds and varieties for production is the initial step in the development of a successful vegetable farming operation. This decision is difficult because Texas producers have many alternatives available to them. Over 40 different kinds of vegetables are grown annually in Texas. Although vegetables are widely adaptable, successful production in a given area will be influenced limiting factors: water, hail, wind, temperature, light, nutrition and markets. Variable levels of limiting factors limit yields or reduce potential for profitability. Therefore, base crop selection on the availability of these factors within a given location and growing season.
Water
Sufficient quantities of high quality water must be available to sustain vegetable farming. As the state’s population continues to increase, less water will be available for vegetable production. Increasing water use efficiency should be one of the major goals of vegetable producers. Vegetable crops require more total water and more frequent irrigation than most agronomic crops. Vegetable water requirements vary from 20 to over 40 acre-inches (543,000 to 1,086,000 gallons per acre) for each growing season, depending on kind of vegetable grown, production location and environmental conditions. Little can be done to reduce water needs of any given vegetable. Plant water use is genetically controlled. However, total volume of water supplied to meet crop needs is influenced by water delivery systems and cultural practices. Irrigation systems are discussed in Chapter V.
Of the irrigation systems available today, drip or trickle systems are most efficient and are best adapted to high value vegetable crops. There are many advantages to drip but ability to place a precise quantity in the exact place where needed is the biggest. This ability enables drip systems to waste less water in the delivery process compared to surface irrigations systems which use ditches, furrows and/or pipes as a delivery vehicle and sprinklers which apply water above the crop.
Water use efficiency can also be increased through effective application scheduling. Several devices and/or techniques are available to aid a vegetable grower in scheduling irrigations. Tensiometers are instruments which measure soil moisture tension which is an indicator of available soil moisture. Irrigation is started when a given moisture tension occurs. The tension at which irrigation begins depends upon soil type and crop grown. Tensiometer measurements should be taken from a depth of one foot for most vegetables. Other effective methods used to schedule irrigations include evapotranspiration rate (ET), a measure of the amount of water used by a crop in a given period of time plus water evaporating from the soil and water vapor transpiring directly from the plant. Weather conditions which effect soil and plant moisture loss are monitored, and a crop coefficient is determined. The Texas AgriLife Extension Service has developed a network around the state to collect and report this data. It can be found on the Web at http://texaset.tamu.edu.
A simplified variation of the ET technique is pan evaporation (a measure of moisture loss by evaporation from a container of water). Pan evaporation can be determined on a farm with a minimal investment in equipment and time. The basic requirements are a rigid container such as a five gallon bucket, a ruler and a rain gauge. Evapotranspiration is estimated by measuring water loss from the surface of an open container (pan) of water. A permanent mark is made approximately one to two inches from the top of the bucket. The bucket is filled with water to the level marked. The amount of water evaporated is measured daily. A ruler is used to measure the difference from the permanent mark to the water’s surface (pan evaporation). Measurements should be taken daily when the crop is grown on light soils or when drip irrigation is used to replenish soil moisture. Weekly measurements may be appropriate with heavy soils and when sprinklers are used to supply needed water. Once a measurement is taken, the water in the pan is brought back to the permanent mark. The quantity of rainfall received is subtracted from the amount of measured loss to give the net loss. Essentially, this is the amount of the water that needs to be replaced. A crop in full vegetative growth usually needs to be replenished at 80% of the pan evaporation level. The amount of irrigation water to apply is determined with the following formula:
Sprinkler applied:
Net Loss x 0.80 = Volume to be Applied
Example: (1″ = 27,154 gallons) x 0.80 = 21,723 gallons or 0.8 inches
Drip applied:
Net Loss x 0.80 x 0.50 = Volume to be Applied
(0.50, a correction factor, normally water only is applied to 50% of the total field area with drip irrigation).
Example: (1″ = 27,154 gallons) x 0.80 x 0.50 = 10,862 gallons or 0.4 inches
No attempt should be made to grow vegetables in Texas without irrigation. Successful dryland vegetable production in Texas is rare. Even sweet potato crops in east Texas (a 50+ inches of rainfall area) require irrigation in most years to be profitable. It matters little how much rainfall is received if it all comes at the wrong time in the growing season. Distribution of rainfall is more important than total volume. Yield reductions can occur if moisture stress occurs during critical growth stages of a crop. Table 33 of the Appendix lists the periods of the greatest water demands by various vegetables. If irrigation water is limited, plant no greater acreage than can be irrigated optimally. Also, chances of success will increase with the production of crops with the least water demands (Table IV-1.)
Water quality and water quantity are equally important. Irrigation water for optimum vegetable growth should contain less than 400 ppm soluble salts. Many Texas wells are becoming more salty. Test well water for salts before planning to utilize them for irrigating vegetables. Knowing the tolerance of vegetables to salinity is essential if marginal quality water is to be utilized. A guide to irrigation water quality is presented in Table 37 of the Appendix. Table 36 includes a listing of crop tolerance to salinity.
Table IV-1. Relative Vegetable Water Needs
Seasonal Water Demand | Crop | Approximate Demand (inch) |
---|---|---|
High | Broccoli | 20 – 25 |
Cabbage | 20 – 25 | |
Cauliflower | 20 – 25 | |
Slicer Cucumber | 20 – 25 | |
Eggplant | 20 – 35 | |
Onion | 25 – 30 | |
Pepper | 25 – 35 | |
Potato | 20 – 40 | |
Pumpkin | 25 – 30 | |
Sweet corn | 20 – 35 | |
Tomato | 20 – 25 | |
Moderate | Asparagus | 10 – 18 |
Pinto Bean | 15 – 20 | |
Cantaloupe | 15 – 20 | |
Cowpea | 10 – 20 | |
Pickling cucumber | 15 – 20 | |
Low | Lettuce | 8 – 12 |
Mustard greens | 10 – 15 | |
Radish | 5 – 6 | |
Spinach | 10 -15 | |
Turnip | 10 – 15 | |
Watermelon | 10 – 15 |
Temperature
Growing season temperature influences crop selection. Vegetables are classified as warm or cool season crops based on their ability to grow within given temperature ranges. Warm season crops are those that produce higher yields under higher temperatures (tomato, pepper, squash); while cool season crops, are those which produce the best under low temperatures (carrot, spinach, cabbage). Table 1 of the appendix defines classification of vegetables based on temperature ranges best suited for production.
Tomato is classified as a warm season crop. High day time temperatures (> 95F) and/or high night temperatures (> 70F) will reduce fruit set, yield, and, induce mottled orange rather than red fruit coloration. Likewise, yields of some cool season crops such as broccoli and lettuce will suffer when temperatures fall below freezing causing discoloration of buds and. Exposure of the fifth to seventh leaf stage onion plants to 38 to 40F for two or more weeks causes flower Stalk development (bolting) which destroys marketability. Therefore, it is extremely important to consider temperature extremes during a growing season when selecting crop species and varieties to produce.
Light
Sunlight intensity (foot candles), sunlight quality (wavelength) and day length (sunlight hours) affect vegetable growth. Most field grown vegetables grow best under a high light intensity. Texas has intense sunlight Intensity is highest in far west and northwest Texas where cloudless days result in over 10,000 foot candles during midsummer. Little can be done to alter light quality under field conditions under field conditions. However, light intensity can be managed by shading, covering crops with row covers, and selecting planting dates which provide the most desirable intensity. For example, crops such as watermelon, cantaloupe and honeydew melons require high light intensity and warm temperatures to produce good growth and high sugar content in their fruit. Therefore, melons are planted in March in the Lower Rio Grande Valley, in March in the Winter Garden, in April in central, east and far west Texas, and, in May on the high plains precisely to have maximum exposure to sunlight and warm temperatures favoring production of high yields of high quality melons. Water requirement and frequency of irrigation increase as sunlight and temperature increase during the growing season in these areas.
Sunlight quality (wavelength) can be managed by mulching soil around plants with certain shades of red plastic which reflects red wavelengths and absorbs blue and yellow wavelengths from sunlight. Infrared (IRT) plastic mulches warm root zone soil faster than black mulch or bare soil and prevents weed growth by not absorbing red and blue wavelengths required for weed growth.
Day length is important in onion production because day length influence onion bulbing rather than flowering as in most other vegetable species. Onion growers manage the influence of day length by selecting varieties with appropriate day length requirements for each of the growing areas. They sow onion seed in October so that seedlings emerge and grow in shortening days (< 10 hours day length) and begin to form blubs in late winter when days lengthen (> 11 hours). Cool temperatures of fall and winter in these three regions are ideal for young onion plant growth.
Day length and light intensity also influences crop water requirements. In general, the greater the intensity of light, the greater the rate of transpiration or water loss from the plant and the greater will be the need to replace the water loss.
Nutrients
Among factors limiting Texas vegetable production, nutrient availability poses the least problem. Fertility of most soils having desirable topography for crop production is manageable. Most fields require nutrient adjustment which is accomplished by applying fertilizers. In order to determine fertilizer requirements collect soil samples at least a month before planting and have them analyzed by a reliable commercial laboratory or in the Extension laboratory at College Station. Request tests for trace elements and salinity in addition to nitrogen, phosphorous, potassium, calcium, magnesium, and pH. Soil test results reveal whether trace element levels are deficient, sufficient, or excessive. These labs can also analyze plant tissue. Such analyses enable growers to monitor crop nutrition during the growing season and to amend his fertilizer program as needed. Table 25 of the Appendix provides information on when and what plant parts to sample for analysis. Growers can also run quick, accurate field tests of leaf petiole sap to monitor nitrogen and potassium levels within vegetable plants during the growing season. In-season test results help growers to determine need, amount and timing for applying nitrogen application at specific growth stages in vegetable growth and development (appropriate forms for soil and tissue analyses by the Extension Service Laboratory can be found at the end of Chapter III or obtained from your local county extension office).
Excessive levels of trace elements can be toxic to plants. Little can be done to reduce excessive trace nutrient levels. Avoid planting soil with excessive levels of these nutrients.
Over fertilization can cause problems in crops. For instance, excessive nitrogen can increase the incidence of gray wall and blotchy ripening of tomato, enhance tipburn in lettuce and soft rot of stored potatoes. Excessive potassium can reduce fruit size and firmness in strawberries; and, leaf chlorosis and stem dwarfing in vegetables can be caused by excessive phosphorous tying up iron and zinc. Over fertilization is easily prevented by managing nutrient levels in the field and crop.
Optimum crop growth and development is attained by timely application and accurate placement of fertilizers. Most vegetables absorb essential nutrients and water from the top eight to twelve inches of the soil profile. Nitrogen, phosphorus and potassium react differently with water and soil, so each must be managed independently. Nitrogen is very soluble in water and leaches downward easily. Phosphorous does not move from where it is deposited. Most Texas loamy sands, sandy loams and clay loams are high to very high in available potassium. Thus, knowing when to broadcast, band, or split-apply nutrients is very important for maximum crop response. To be certain that soil fertility is optimum for early growth of seeded and transplanted vegetables apply ¼ to ½ of the nitrogen (sandy loams to clay loams, respectively) plus all phosphorus and potassium before planting. For many transplanted vegetables and potato seed pieces, preplant fertilizers are applied broadcast and worked into soil as raised beds are formed. Alternatively preplant fertilizers can be disked in for non bedded culture in sandy soils under center pivots.
The most effectively managed fertilizer programs to obtain maximum early and total yields of most vegetables (especially those planted in cold soils) consist of banding phosphorus beneath the seed row or transplant ball and split bands nitrogen and potassium on either side of seed or transplant row. As mentioned earlier, apply ¼ to ½ of the nitrogen (light and heavy soils respectively) and all of the phosphorous and potassium prior to planting. Band placement is critical. Phosphorous should be placed approximately two inches below the seed row or transplant root ball for maximum benefits. Phosphorous banding has also been shown to reduce the total amount needed without adverse yield effects, and, to reduce tie up of minor elements such as iron and zinc. The remainder of the nitrogen is then applied as a top or side dress application.
Correct amounts of preplant nitrogen are seldom sufficient to satisfy total requirements of long season vegetables. The increased nitrogen leaching potential of lighter soils will dictate more frequent top- or sidedressing to maintain optimum growth and yield. Band placement of nitrogen is also critical. Nitrogen fertilizers are salts which affect plants in the same way as do sodium salts. Too much nitrogen placed too close to the seeds or root mass can result in crop injury or death. Therefore, never apply more than 20 lbs actual nitrogen per acre. Band the nitrogen approximately 4 to 6 inches to the side and below the seed row at planting, and, 6 to 8 inches to the side and below young seedlings.
Another mean of applying a sidedressed or supplemental nitrogen is through irrigation water or fertigation. The most efficient fertigation method is with drip irrigation. Fertilizer is easily applied and uniformity distributed. Many growers have obtained exceptional results by applying 4 to 5 lb N/A (20 ppm) with each irrigation until the onset of crop maturity. Such a practice is well adapted to use on light soils. Checking leaf petiole nitrogen is a good way to monitor correctness of application rates and timing. Fertilizers must be soluble in order to be injected into an irrigation system. Those fertilizers in a dry granular or crystalline form must be dissolved in water to form a solution. Once the desired concentration is obtained the solution can be injected. The fertilizer injection should occur well upstream of any branching on the flow and in front of the filter. This will allow thorough mixing of the solution into the irrigation water and help to prevent contamination of the system. All of the drip lines should be pressurized and filled with water prior to injection. Once the injection is completed, the drip system should continue to run for a while to flush the lines. Nitrogen is the most commonly applied nutrient through drip systems. Table IV-2 lists the nitrogen requirements of vegetable crops when fertigation is practiced.
Table IV-2. Nitrogen Requirements for Fertigation of Vegetable Crops
Crop | Growth Stage | N required (lb/A/week) |
---|---|---|
Broccoli | Early Growth | 5 – 101 |
Midseason | 10 – 20 | |
Button Formation | 15 – 30 | |
Head Development | 10 – 20 | |
Cucumber | Vegetative Growth | 5 – 10 |
Early Flowering/Fruit Set | 10 – 20 | |
Fruit Bulking | 10 – 15 | |
First Harvest | 5 – 10 | |
Lettuce | Early Growth | 5 – 10 |
Cupping | 10 – 20 | |
Head Filling | 15 – 30 | |
Melon | Vegetative Growth | 5 – 10 |
Early Flowering/Fruit Set | 10 – 20 | |
Fruit Bulking | 10 – 15 | |
First Harvest | 5 – 10 | |
Pepper | Vegetative Growth | 5 – 10 |
Early Flowering/Fruit Set | 15 – 30 | |
Fruit Bulking | 15 – 20 | |
First Harvest | 5 – 10 | |
Squash | Vegetative Growth | 5 – 10 |
Early flowering | 10 – 20 | |
First Harvest | 5 – 10 | |
Tomato | Vegetative Growth | 5 – 10 |
Early Flowering/Fruit Set | 15 – 20 | |
Fruit Bulking | 10 – 15 | |
First Harvest | 5 – 10 |
1 Higher values represent fertilization needs in soils with small amounts of residual nitrogen or where higher temperatures causes rapid plant growth
Source: Hartz, T. K. 1993. Drip irrigation and fertigation management of vegetable crops. Department of Vegetable Crops, University of California, Davis
When practicing fertigation, measures must be taken to prevent contamination of the soil and the water supply. Contamination can result if the irrigation pump shuts down during the operation of the injection equipment causing fertilizer to be injected into the water supply or if the injector stops while the irrigation system continues enabling irrigation water to flow into the fertilizer tank and to overflow onto the soil. Several devices are used to prevent the above such as vacuum breakers (atmospheric and pressure types) and, single or double, check valves.
Phosphate fertilizers injected into a drip system may cause chemical or physical precipitate clogging of emitters. Phosphorous is also rapidly tied up and does not move readily into the soil profile. Therefore, injection phosphorous into a drip system is not very desirable.
Caution should also be taken if potassium fertilizers are mixed with other fertilizers. Potassium, like phosphorous does not move readily through the soil profile. Potassium chloride is the usual form of K injected into drip systems but for chlorine sensitive crops, potassium nitrate may be preferable.
Where readily available, spreading two to three tons of compost per acre every year and growing soil building crops help to stabilize the soil ability to hold and release nutrients optimally as needed by vegetables. Fall sown Elbon rye (seeded in September or October) is an excellent cold hardy soil building crop which develops deep and spreading roots in fall and winter. The crop should be well supplied with nitrogen and water, and mowed to keep it to a manageable height. The crop should be turned under in early spring before extensive top growth hampers tillage and seedbed preparation. Remember to leave very narrow strips not more than a foot wide every four to eight rows to provide valuable windbreaks.
Markets
When most people refer to limiting factors which must be managed to succeed in crop production, they usually discuss pest, climate, soils, varieties, equipment and labor. However, in Texas the major factor limiting profitable vegetable production is market availability. It matters little if all of the physical factors are favorable in an area if market availability is not. Significant increases in profitable vegetable acreage can only occur if additional markets are accessed or developed. Therefore, crop selection should be based on ability to profitably market the produce. The ultimate goal of every commercial enterprise is to sell its produce for a profit. It is imperative that growers know what the crop market potential is prior to investing money and time in production. After this is determined, the decision as to which crop to produce becomes clear. Produce only those for which a market exists. Tables I-1 and I-2 of the Introduction depict normal marketing season for Texas grown fruits and vegetables plus availability of such commodities from Mexico.
Variety Selection
Once the decision which crops to grow is made, the variety selections must be made next. Always select adapted varieties having the highest levels of disease and/or insect resistance, good horticultural characteristics and market appeal. Variety suggestions for the major Texas vegetables crops are presented in Table 15 of the Appendix.
Site Selection
Three major points should be considered in selecting a field for growing vegetables: site topography, soil type, and water availability (quantity and quality).
Topography refers to the physical layout or characteristic of the field area. Elevation, contour, soil depth, water and air drainage patterns as well as the presence or proximity of rock out cropping and trees can significantly influence production. An optimum topography for a vegetable field is one that is nearly flat to slightly sloping, well drained and free of low spots, rocks or trees. Fields of this type are desirable because cultural practices such as cultivation, irrigation, pest management and harvesting are performed more efficiently on level unobstructed land.
Soil type refers to the physical makeup of the soil. Basically, soils are composed of decomposed mineral matter (sand, silt, and clay) and decomposed organic matter (plant residues). The optimum soil conditions for most vegetables consist of a deep, friable, well drained, sandy loam. Clays and other heavier soils are not well suited for vegetable production due to poor aeration, drainage, nutrient liberation and restrictive root growth characteristic of such soil types.
Soil type is not as important as soil management for vegetable production. Soil leveling and the use of high beds to facilitate drainage may be necessary with heavier soils. Light sandy soils are more prone to nutrient leeching than the heavier clay soils. Therefore, split nutrient applications rather than total preplant nutrient application is suggested for these types. When earliness is more critical than total yield, a light sandy location is more advantageous. Whereas, when total yield or moisture retention is the primary concern, selection of a site with a heavier soil may be more desirable.
Few vegetables are produced as dry land crops in Texas. Therefore access to an adequate supply of good quality water is essential to optimize yield and quality. Availability of water during key growth stages can often determine success or failure of a crop. Critical water usage periods are listed in Table 33 of the Appendix. Guidelines for supplemental irrigation and irrigation systems are discussed in Chapter 5. Topography and ideal soil type cannot offset the absence of an adequate supply of good quality water.
Land Preparation
Most vegetables have small to medium size seeds. Consequently, proper seed bed preparation is important. Optimum seed beds have mellow soils comprised of fine sized particles, free of clods, weeds and previous crop residue. Such seed beds enable good soil to seed surface contact to soil which is required to allow uniform water absorption, germination, and seedling emergence.
Several steps are required to develop mellow seed beds. The first step is deep moldboard plowing. A field which has not been planted to any crop or not to vegetables should be plowed to a depth of 12 inches or more. For example, deep plowing is a must for a field of native vegetation or pasture grasses being put into vegetable production. Deep plowing will promote complete decomposition of plant material to occur. If the residue is excessive, deep plow in the fall. This will allow sufficient time for the decomposition to occur prior to spring planting. Deep fall plowing is also advantageous where soil freezes. Freezing and thawing improves the soil structure.
If the field site selected has been continuously cropped, deep plowing or turning of the soil every other year may suffice unless a large amount of crop residue must be turned under. Deep chisel point plowing done in the off years can improve or maintain the soil’s physical condition by penetrating and fracturing compacted soil. This is desirable for the prevention of a plow pan or a compacted soil layer forming at the depth of the plow blade. Such formations can cause problems with respect to downward water percolation and normal root development. Seeding directly over chisel marks permits melons, pumpkin and tomato roots to penetrate deeply into the soil profile.
Never plow nor disk water saturated soil. Let wet soil drain until it can be gently squeezed without getting your hand wet and the plow slice crumbles as it is turned over. If soil crumbles readily after being compressed together in your hand, it is dry enough to work. Working wet soils cause clod development. Colds will not pulverize to create a good seed bed.
Discing in two directions should follow deep breaking or plowing. Tandem and/or offset harrows work well. If the field is to be furrow irrigated, form rough raised beds using bed listers. Best height of bed is determined by soil type, intended crop and irrigation method. Raised beds six to eight inches high work well for most areas of Texas. Raised beds ensure good drainage of excessive moisture, early soil warming, rapid drying of soil surface, improved soil aeration and less chance of soil borne diseases. This is especially true on heavier soil types. Generally, the heavier the soil, the higher the bed should be constructed.
If soil moisture is insufficient for planting after bedding, preplant irrigate to replenish moisture to field capacity. Once the soil sufficiently dries, the rough beds should be reworked using a rolling cultivator or a power rotovator. Do not beat soil to powder. Rotovator mellowing will improve the soil structure by breaking up any remaining clods and kill weed seedlings which emerged as a result of the preplant irrigation. Final seed bed preparation step entails firming and/or shaping the beds with a bed roller or a sled type shaper. Seed beds are similar to the foundation of a building. Mistakes during development are seldom overcome. In all these operations greater grower skill comes with experience and working through trials and errors.
Markets
Regardless of the kind of market, buyers of fresh vegetables want continuous supplies of high quality produce. To sustain long term profit, a grower must build a good reputation over many years in succession for consistently high quality and reliable volume. Ideally buyers for supermarket chains want to be assured of at least six to eight weeks of continuous supply of a given volume of a vegetable from a grower or shipper before they will agree to buy from that farming operation. In many instances it may be necessary for growers schedule plantings to provide a continuous supply of a given volume of a commodity. For example, a given supply of a given volume of sweet corn from mid-June through October can be achieved by suing a heat unit (Growing Degree Days) system for scheduling planting.
The use of degree days has become a standard practice in the processing industry for crops such as sweet corn and peas. The system utilizes the accumulation of temperature above the base temperature below which no growth is expected. It is calculated as follows:
2
The base temperature is that below which appreciable growth ceases. The base temperature for sweet corn is 50F.
Crop Establishment
There are three basic methods of establishing vegetable crops in field situations: direct seeding seeds; transplanting seedlings, and, planting vegetative plant parts. Choice of the crop establishment method will depend upon the crop, the market windows, cost and availability of seeds, plants and specialized equipment. Some crops can be established commercially only by using a specific technique. For example, potatoes must be established with seed tuber pieces. If an early market is the prime concern, use of transplants may result in harvest 1 to 2 weeks earlier. Seed cost may so high that direct seeding is prohibitive. Conversely, time required, plant cost, labor and/or specialized equipment required for transplanting may render this technique uneconomical for some crops.
Texas vegetable producers often have difficulty achieving desired plant stands. Many factors may contribute to this situation including soil borne pathogens, insects, weed competition, soil crusting, and climactic conditions. In order to obtain an optimum stand, one or all of the above variables must be managed to some extent through the use of specialized cultural practices.
Soil borne disease and insects are difficult to control. A combination of several practices may be used to obtain economic control depending upon the type and/or seriousness of the problem (Refer to Chapter VI for insect control measures and Chapter VII for disease control measures). Weed competition can also cause serious stand reductions Weed control measures are discussed in Chapter VIII.
Time and care invested in seed bed preparation is well spent when trying to produce a vegetable crop. Level, well pulverized, firmed but not compacted planting beds are essential for small seeded crops such as carrot and broccoli. Firm, mellow beds enable precise seed placement. Seeding into cloddy, uneven beds causes uneven seeding depth poor seed to soil contact. Uneven fields also do not wet uniformly during irrigation. Uneven soil moisture causes more plant skips in the seed row, uneven emergence and variable plant maturity, and poor weed control. Inefficient production and increased management stress are consequences.
Focus attention on careful seed bed preparation and seed placement where vegetables are to be mechanically harvested. Today most crops grown for processing in Texas are mechanically harvested. The mechanical harvesters perform well only in fields having even seed beds, uniform stands and consistent plant size and crop maturity.
Over pulverized seed beds also can cause problems. Heavy rains or overhead sprinkler irrigation can cause these beds to crust. Most small seeded vegetables do not emerge well through the crust. Seedlings often are unable to break through crust. Reduced stands are the result.
Some soils are more prone to crusting than others. Clay loams and clay soils crust easily. Clay particles bond to each other strongly forming plates. When wet clays are dry they form crust at the soil surface. The faster the drying occurs, the greater the crusting problem. Low relative humidity, high temperatures and strong winds accelerate crusting. Pay special attention to keeping soil moist in the seed zone with soils prone to crusting. Keeping this area moist reduces the effect of crusting and enables unimpeded seedling emergence. Sprinkler irrigation compounds crusting and stand problems by pounding clay particles into tighter layers, which often trap seedlings before they can emerge and/or stick emerging seedlings to the soil surface. Well managed drip or furrow irrigation favors stand establishment because water seeps into the bed soil without impacting the bed surface. Applying more water than is necessary to rewet the seed zone keeps soil too cold and slow germination.
If soil tends to crust, consider the use of anticrustants, soil conditioners, and/or moisture loss retardants. These materials reduce crust strength, suppress moisture loss and/or increase the water holding capacity of the soil in the seeding zone. Sources of these materials are shown in Table 18 of the Appendix. Adding organic matter will also reduce surface soil crusting.
Direct Seeding
Approximately 85% of the vegetables grown in Texas are established by sowing seed directly into the field soil where the crop will be produced. Care should be taken to adjust and operate seeders to firmly position seeds at optimum depth. Seeds planted too deep often do not emerge adequately to produce a desirable stand. For example, bean and pepper seedlings are easily obstructed in their upward thrust and fail to emerge is planted too deep. Because most vegetable seeds are relatively small, they do not have sufficient emergence strength to push through deep soil. This is especially true under cold, wet soil conditions. Seeds planted too shallow often dry out too rapidly causing poor germination.
Optimum planting depth for most vegetables is three times its width. For example, bean seeds are generally ¼ inch in width. Therefore, bean seeds should be sown ¾ inch deep. Appendix Table 4 presents the optimum suggested planting depths for the most common vegetables grown in Texas. Vegetable size is significantly influenced by plant density. Exercise care in accurate seed spacing. Optimum plant spacing for selected vegetables is presented in Table 3 of the Appendix.
Over or under planting causes problems. Nothing can be done for an under planted field, whereas, over planted fields can be manually thinned to the desired stand. Thinning is, time consuming and expensive. Additionally, high performing hybrid varieties with costly seed are gaining in importance in the vegetable industry. Seed cost for hybrid varieties dictates that seed germination and seedling emergence is maximized by precision planting.
The advent of precision planters which achieve desired stands has enabled planting-to-stand to become a common practice. There are several excellent precision planters available on the market today. Sources for these are presented in Appendix Table18. Precision planters are expensive, but savings from reduced seed and thinning costs soon pay for them. Careful bed preparation is absolutely essential to profitable use of precision seeders.
Types of Precision Planters
Belt type: Delivery system includes belts with circular holes punched to accommodate the desire seed size to be planted. Holes are spaced along the belt at a specified distance to approximate field seed spacing (Example: Stanhay seeder.)
Plate type: Delivery system includes a notched horizontal or vertical plate which is used to space seed (Example: John Deere 33 or Earth Way.)
Vacuum type: Delivery system draws seed against holes in a vertical plate which is agitated to remove excess seed. Field spacing achieved with a combination of gears and number of hoes per plate (Example: Gaspardo and Monosem.)
Sources for precision planters are given in Table 18 of the Appendix.
The use of hybrid varieties and precision planting may not be the total solution to optimum stand establishment. Some vegetable seeds work better than others in most precision planters. Round seed are easier to singulate and place properly than oblong or flat seed. To overcome this problem, seed coating has been developed. Seeds are coated with clay materials which give them a rounded appearance. The use of coated seed can reduce the problems with accurate seed placement encountered with precision planters.
Seed coating does not always assure rapid and uniform emergence under adverse conditions. Seed enhancement has been found to encourage rapid germination and uniform emergence. Simply stated, enhanced seed has been treated in such a manner so as to activate the germination process but does not allow germination to occur until seeds are placed in a moistened rooting media. If the need for a uniform crop exists, precision planting of coated, enhanced seed is suggested. If a specific harvest season requires a crop to be sown in less than desirable conditions, coated, enhanced seed can be of value. Not all vegetable species are being enhanced by the seed companies at this time. This is unfortunate because enhanced seeds germinate faster and emerge more uniformly under most conditions and add crop value by insuring acceptable stands of high priced hybrids.
Transplanting
There are five primary advantages to transplanting:
- Enhanced earlier harvest
- Reduced impact of adverse environmental conditions during the early seedling growth
- Reduced seed quantity needed for crop establishment
- Enhanced plant stands and faster maturity
- Eliminates thinning needs
An increasing number of Texas vegetables are commonly grown from transplants including: broccoli, cabbage, cauliflower, Brussels sprout, onion, tomato, pepper, eggplant, celery, cantaloupe, honeydew and watermelon.
Use of transplants for hybrid tomato, pepper and eggplant is essential for profitable commercial production and timely marketing of these vegetables in Texas. These vegetables are warm season crops. However, flowering and fruit set must occur before it gets too hot. For maximum yields from these crops, flowering and fruit set needs to occur before night time temperatures exceed 72 to 75F in combination with daytime temperatures above about 92F. Some varieties of these vegetables will set some fruit above these temperatures, but excessive blossom drop will occur. Normally there is a 2 to 3 week period in which favorable conditions prevail for optimum fruit set. Direct seeding after danger of frost is over will result in late blooming during high, unfavorable temperatures. This will result in an excessive blossom drop and poor fruit set which reduces growers’ profitability.
High seed cost and poor germination ability under field conditions renders transplanting a must for the establishment of seedless watermelons.
Transplant production
- Sow appropriate quantity of seed in sufficient time to grow a desirable plant prior to the anticipated planting date (Table 9 of the Appendix).
- Sow small seeded vegetables, e. g. tomato and broccoli, into seeding trays, flats or plug trays filled with a standard rooting mix (ex. Jiffy Mix, Redi-Earth, Sunshine Mix).
- Sow seed rows in flats for easy uprooting and separation for transplanting
- Base seeding depth on seed size (Table 3, Appendix).
- At full cotyledon expansion, pull seedlings carefully and replant in final growing containers filled with rooting mix (1 seedling/pot.)
- Sow larger seeded crops such as watermelon directly into final growing containers (2 to 3 seeds/pot and thin to 1 at the cotyledon stage).
- Pot or cell size depends upon vegetable species (larger cell sizes for larger seeded vegetables).
- Maintain the greenhouse or growing structure temperature at 60 to 65F day and 50F night for cool season vegetables and 75 to 80F days and 60 to 65F nights for warm season vegetables
- Maintain good ventilation during the heat of the day. Use evaporative coolers to reduce daytime temperatures below 85F if possible.
- Harden plants for one week prior to anticipated field setting by exposing to lower temperatures and reduced watering. Note – Approximate time to grow a field ready transplant is shown in Table 6, Appendix.
- Field grown bare root transplants possible in nurseries protected by wind breaks and the use of row covers.
Purchasing Transplants
Most commercial growers find it more cost effective to purchase transplants on a contract basis than to grow their own. When purchasing transplants; order six to eight weeks in advance of the anticipated field planting date. Always purchase plants from an established reliable nurseryman. Known transplant growers are listed in Appendix Table 17.
Troubleshooting Transplants
Tall, Spindly Plants: Insufficient light; crowded growing conditions; excessively warm temperatures.
Slow Growth: Lack of fertility; insufficient light or poor root growth due too over watering; use of heavy potting soils.
Chlorosis (yellowing): Deficiency of nitrogen; water logging.
Damping off: Poorly drained potting soils; excessive watering. Damping off is caused by fungal diseases of young seedlings. The primary symptom of this disease is a water soaked appearance on the lower seedling stem causing plants to fall over and die. A fungicide drench over the seedlings and improved air circulation is suggested.
Transplanting Keys
- Thoroughly water plants 12 to 14 hours prior to transplanting.
- Prevent roots from exposure to sunlight after removing from growing structure and prior to field setting.
- Place transplants in a hole deep enough to cover the entire root ball or peat pot (exposed ball or peat pot will cause excessive moisture loss and possible plant death).
- Apply 8 oz water or starter solution/plant (Example: 3 lb of dry high phosphate fertilizer or 2 pt of liquid formulation such as 0-46-0, 11-55-0 etc. to 50 gallons water, or 1 lb regular commercial fertilizer 5-10-5 per gal water for stock solution. Mix 5 gal stock solution per 45 gallon of water. This reduces transplant shock and aids new root establishment.
- Best results obtained when plants are set in moist soil. If soil is dry, irrigate immediately after transplanting.
Vegetative Propagation
Vegetative propagation entails the use of a plant part to regenerate new plants or to establish a new crop. In Texas, only two of the major vegetable crops are established in this manner, Irish potatoes and sweet potatoes. Irish potatoes grown for replanting are cut into 2 oz seed pieces. Each seed piece must contain at least one vegetative bud (eye) to enable a new plant to develop once the seed piece is planted. Cut tuber seed pieces are healed over by storing them at high temperature (85 to 90F) and high relative humidity (85 to 90%) for one to two weeks prior to planting. Whole, small potatoes (B size) can also be used to establish the crop. Sweet potato mother roots are bedded in plant beds where they sprout new stems called slips. Slips are pulled at 6 to 9 inches in height. These slips are then planted into the production field.
Crop Stress Reduction
Crop stress is a condition which results from the impact of any environmental factor causing plants to deviate from their optimum growth and development rate. Dealing with the effects of stress caused by unstable Texas weather conditions is the most difficult challenge facing vegetable growers. Many cultural practices have been developed to alleviate or counteract the influence of stressful conditions. Proper selection of cultural practices can help to ensure development of a highly successful vegetable production operation.
Seed Bed Manipulation
As a rule, the slower the speed of germination and seedling emergence the greater is the chance of stand loss due to crusting, disease or insects and weed competition. Cold, wet soils retard germination and emergence. Plant death and disease resulting from action of these stress factors can be reduced by allowing soils to reach optimum seed germination temperatures prior to seeding. The influence of soil temperatures on germination can be found in Table 4 of the Appendix. Little advantage is derived from planting seeds into soils which are too cold or too hot. In most cases, even if germination and emergence occur, severely reduced plant growth often results in yield reductions.
Cultural practices can be selected which warm the soil and promote germination if seeds must be planted in cooler than optimum temperatures. Seed bed orientation and configuration have been successfully employed for this purpose. South sloping, east west running beds promote rapid warming by exposing maximum bed surface to the sun’s rays and serving as a barrier to cold north winds (Figure IV-1). Sloping beds enhance drainage of excess moisture and allow soluble salts to move up the slope and out of the seed and root zones. Applications of black liquid spray and/or petroleum based spray mulches to bed surfaces after seeding increases bed warming and enhance germination and emergence. Similar results can be derived by direct seeding through black plastic mulched beds.
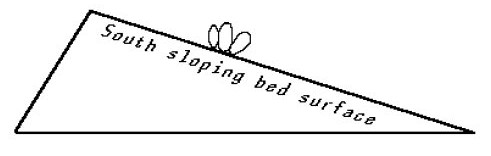
Figure IV-1. Cross Section of a South Sloped Bed for Added Soil Warming
High soil temperatures retard or prevent germination of some vegetable seeds such as lettuce and spinach. These crops can experience heat induced dormancy which inhibits or reduces germination. In Texas, high soil temperature is often accompanied by drought conditions. Seed bed configuration will be valuable in these situations. Depressional planting, sowing seeds in depressions or trenches 3 to 4 inch deep by 5 to 10 inch wide on raised beds enables moisture to drain down to the seed zone. This cools the soil and maintains moisture longer to enhance germination. The walls or sides of the depressions block out hot drying winds, a major problem with stand establishment during late summer, early fall planting season.
Seed bed manipulation will ease plant stress caused by high salt content in soils or irrigation water. Because salts tend to percolate upward to the highest point on the plant bed, planting on either side of a peaked bed enables stand establishment in otherwise unusable areas (Figure IV-2). Similarly, planting at the bottom of trenches on raised beds can also alleviate the problems of high salt content (Figure IV-3). These beds cause salts to move up above the seedling zone. Using transplants is additional method for optimizing plant stand during unfavorable seed germination conditions.
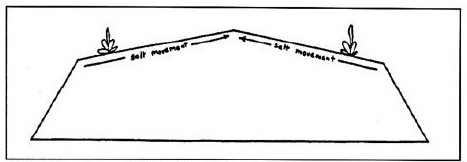
Figure IV-2. Cross Section - Peaked bed for salinity management.
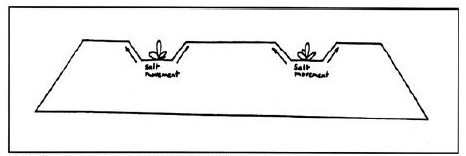
Figure IV-3. Cross Section - Trenched bed for salinity management.
Windbreaks
Growers often do not realize how wind hurts their vegetable crops. Researchers recorded lower yields from vine movement of greenhouse grown cantaloupes compared to yield from unmoved vines. Under field conditions, wind can do more than move plants. Wind speed over 25 mph sandblasts and desiccates leaves. Combined effects of wind whipping across fields reduce yields. Reduce impact of wind on vegetable health by growing windbreaks in fields exposed to wind.
Wisely spaced, timely planted, windbreak plants are protective and cost effective barriers. Choice of temporary or permanent windbreaks depends on available planting and plant species. Tall growing trees planted on the windward side of a field offer a permanent solution. Trees develop slowly and require considerable space. Windbreak trees are available at low cost from the Texas Forest Service. Temporary windbreaks of tall-growing grasses, e.g., Elbon rye and Tascosa wheat, planted sequentially across the field complement permanent windbreaks located along the edges of fields. Trees provide excellent protection from spring west winds for transplant nurseries, early planted crops on mulch or under row covers, and vine crops which suffer whiplash in wind. Semi-permanent switch grass windbreaks and narrow rows of fall planted Elbon rye are well adapted to fall seeded onions, early spring planted potatoes, spinach, cold tolerant sweet corn and al vine crops.
Establish a temporary windbreak system well in advance of the intended crop.
Some producers utilize row covers, cages and/or trellises to overcome the effects of the wind or species adapted to this type of culture.
Windbreaks and Nurse Crops
Windbreaks and nurse crops are essential to protect vegetable crops from wind and hail. Windbreaks have been found to increased cotton yield 45% and onion yields 38%. Farmers consider cotton and onion to be wind tolerant crops, and they are wind tolerant if you are talking about plant survival. Windbreaks permit vegetable plants to grow faster and larger by lowering rate of water loss from soil and leaves and increasing air and soil temperatures near the soil surface in spring. Developing compatible windbreak/vegetable crop systems requires deep thought and detailed planning, but consistent crop success is the payoff. Windbreak alternatives include Elbon Rye, Tascosa wheat, G-1990 sorghum, tall oats, switch grass, tall sunflowers, corn and sweet corn. The difference in feet between windbreak height and crop height multiplied times 10 gives the lateral distance across rows receiving benefit (slower wind speed).
The choice of windbreak species for use is based upon the area of the state, the season the crop is to be grown and the type of crop produced. Several windbreak crops adaptable in certain regions are available to Texas Growers. A few of the common ones are:
- Fall planted Elbon cereal rye in East Texas, Winter Garden area, and northward through the Panhandle
- Emmer speltz wheat in the Rio Grande Valley
- Tascosa tall growing wheat in the High and Rolling Plains
- Spring planted Headless Wonder or Do More sterile grain sorghum for all regions
Although cropping patterns often dictates how close windbreaks can be established, they should be planted as close together as possible. With certain crops, such as tomatoes or melons, where wide bed spacing is normally utilized, establishment of windbreaks between each plant row affords maximum benefit. Such arrangements can moderate the microclimate to promote earliness as well as reduce crop losses. To accomplish this, the entire field is planted with a cereal grain in the fall and stripes are removed in the spring in areas where a crop is to be planted. Strips can be removed by rototilling, disking, and/or spraying with a contact herbicide prior to rototilling or disking. The remaining cereal grain crop should also be sprayed with a grass selective herbicide or a desiccant type herbicide or a systemic such as Roundup before seed maturity occurs in the windbreak species that will enable the residue to remain standing. This will afford wind protection for the season but remove competition to the vegetable crop.
Some wind speed reduction from windbreaks will be experienced over a distance equaling 10 times the difference in height between the windbreak and crop. For maximum benefit, windbreak rows should be planted at a distance apart equaling 5 to 7 times the normal height of the windbreak species. For example, if a species normally grows to a height of 5 feet, higher than the vegetable crop, windbreaks should be established 25 to 35 feet apart. It may be necessary to establish temporary windbreak system well in advance of the intended crop. Because of the amount of land area taken out of production for their use, many producers are reluctant to use this valuable cultural practice. Adverse effects of wind can also be overcome with the use of row covers, cages, and trellises. Strong rye and tall wheat windbreaks protect onions, peppers and other vegetable from hail damage as well.
Mulches
Mulch is any material applied to the soil surface of a plant bed to modifying the microclimate just above or below the soil surface. Depending upon the material used and the time of the year, mulches can successfully suppress weed growth, reduce soil moisture loss, reduce fertilizer leaching, overcome unfavorable soil temperature, manipulate insect populations and/or manage disease problems. Mulching increases yields, induces earliness, and improves quality of most vegetable crops.
Mulch materials can be classified into organic or synthetic. The first mulch used was the organic types consisting of grass straws, leaves and grass clippings. The more effective organic mulch materials are wheat straw, hay, and rice hulls. Benefits derived from organic mulches are; soil moisture retention and addition of organic matter to the soil after crop harvest. Major disadvantages of organic mulches are reduced soil warming if applied too early in spring and require addition of nitrogen fertilizer to compensate for the soil nitrogen used in decomposition of plant material. Additionally, application requires considerable labor.
More recently, the use of synthetic mulch (plastic films, aluminum foils, paper products) has gained wide spread acceptance. Of these, the plastic mulch is by far the most widely used. Plastics are the most versatile mulches and their application is fully mechanized (See Appendix, Table 38 for applicators sources). They also provide better weed control, enhance soil warming, and, can serve as an aid to fumigation. The two major disadvantages of plastic mulch are; cost of the materials, and, disposal. Although there are both photo and biodegradable mulches available, their use has been somewhat limited due to the unpredictability of their breakdown. Consequently they must be removed from the field and disposed. This operation is costly and most landfills will not accept used plastic. Fortunately, some recycling companies are being to accept used plastic mulch.
Because there are many mulching materials from which to select, growers should select the ones which best meet their needs. If large acreage is involved, then the speed and ease of application is the primary concern and the choice is limited to plastics. If crop earliness in the spring is the major concern, select mulches which rapidly warm the soil should.
Clear plastic provides the greatest warming of all mulch materials. However, because weed seeds rapidly germinate under clear plastic, it should not be used without an effective herbicide. New brownish tinted plastic named infrared transmitting (IRT) plastics do a fairly good job of preventing weed seed germination. Although black plastic does not warm as efficiently as clear, it does prevent weed growth by blocking out light and would be the best choice in most cases where earliness is desirable. Conversely, if a fall crop requires planting into excessively hot soils, reflective mulch, such as aluminum foil, white plastic or paper, are more advantageous. Because aluminum foil is extremely difficult to handle, many growers have begun to spray paint black plastic with aluminum paint. Needless to say, this is a very expensive technique. Recently, plastic mulches, which are black on one side and white or silver on the other, have been introduced. This allows selection of the color needed depending upon the season. In small scale production, loosely applied wheat straw mulch is a very effective means of maintaining soil temperature between 70 and 75°F.
Reductions in soil moisture loss of 10 to 50% can be achieved depending upon mulch material. Greatest reduction in moisture loss is accomplished with plastics. However, important consideration with respect to soil moisture is the content at application, the size of the bed to be mulched and the soil type. Synthetic mulch is impervious to water so rainfall is shed from the mulched bed surface. Therefore, when using these materials, a preplant irrigation to fully wet the soil profile prior to mulching is advisable. This is especially true when wide beds are constructed, such as those used in melon and tomato culture, and when mulching lighter soils. Generally the lighter (sandier) the soil, the poorer is the water movement laterally. As a result, water received or applied to the furrows between the mulched areas does not always move to the bed centers where the plants or seeds are to be placed. Therefore, the use of more porous mulches developed from plant residues may be appropriate in situations where rainfall is a valuable source for crop moisture or on very sandy soils that are to be sprinkle irrigated. If plastic mulches are to be used in these situations, they should be used in conjunction with drip irrigation where the drip line is applied prior to mulch application.
The ability of plastic mulches to shed water from the bed surface is an asset during raining conditions. These mulched beds tend not to become water logged as easily as bare soil or beds mulched with organic materials.
As indicated earlier, mulching has been found to be beneficial in managing disease and insect problems. Reflective mulches such as aluminum coated plastics and paper are known to reduce aphid buildup. This benefit has lowered the incidence of aphid transmitted viruses. Due to the intense heat buildup under clear plastics, reductions in the incidence of several soilborne pathogens have been noted. This process known as solarization is gaining importance in disease management programs. Solarization is accomplished by mulching beds with clear plastic in early to mid-summer and allowing the plastic to remain there until just prior to planting in late fall or early spring.
Another technique that centers on the use of plastic mulch is one referred to as rainfall capture (RFC). In this technique, seed beds are configured in a manner similar to that previously described for depression planting and illustrated in Figure IV-3. After the seed bed is reconfigured, plastic mulch is laid over the bed surface in the conventional manner. Holes are punched in the plastic at 3 to 4 feet increments down the bed over the trenches. Soil is placed over the holes in the mulch to cause the plastic to stretch and form a lining of the trenches. The holes in the mulch are now at the bottom of the trenches and serve as avenues of entrance for rainfall and other forms of moisture into the bed profile (Figure IV-4). Consequently, sprinkler applied water, rainfall and other forms of moisture is concentrated in the trenches and channeled into the soil profile rather than shed from the mulched surface into the furrow between the beds and out of the field. Seed and/or transplants can be planted in the area between the trenches.
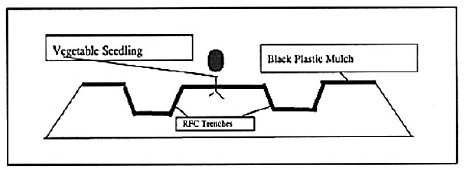
Figure IV-4. Cross Section - Rainfall capture bed
When RFC is established for a period of time prior to planting it also offers the potential to reduce the need for preplant irrigation often required to fill the soil profile with moisture. Starting the planting season with the soil profile filled with moisture is vital to successful vegetable production. This is especially important when using drip irrigation and/or plastic mulch on the lighter soil types. Such practices are not conducive to catching up in moisture deficit situations. RFC established prior to a high rainfall probability period preceding anticipated crop planting date enables sufficient moisture to be captured and stored for use in seed germination and seedling establishment. For instance, RFC established in late August through Mid October in South Texas provides an opportunity to capture normally occurring fall rains and store the moisture for use by a spring planted crop.
The importance of capturing and storing moisture is realized when considering the required water input to affect a particular plant response. From this standpoint the least efficient use of applied water occurs when a preplant irrigation is required to fill the soil profile in order to facilitate seed germination. Under laboratory conditions, sufficient seed to plant one acre of a crop such as pepper or cantaloupe can be accomplished with one pint of water. However, under field conditions, it is not unusual to apply ¼ to ½ million gallons of water to accomplish this process.
In recent years, colored mulch also has been shown to influence crop performance. Certain shades of reds have been reported to increase yields of certain crops. Silver or reflective mulch has also been reported to repel aphids and other insects, whereas, yellows tend to attract insects. Just as with most precision farming equipment, in order for a mulch applicator to function properly, good seed beds are a must. Slightly raised beds with well pulverized soils are the most desirable. This allows easy covering of the plastic edges and enables the plastic to be securely anchored. If a good cover is not achieved, plastics may be blown out of place by excessive winds. Additionally, soils free of clods are a must in order to prevent tearing of the mulch as it is being stretched across the bed surface by the applicator. The better is the soil to plastic contact the better will the results obtained from the use of mulch. Therefore, well prepared seedbeds are essential.
There are three basic operations needed to accomplish this: 1) pulverizing and bedding the soil; 2) shaping or pressing the beds; and 3) laying the plastic. These operations can be done separately or as a combined process. For best results plastic mulch should not be applied when air temperature is below 75°F. If applied under cool or cold conditions, plastic stretching capability is reduced. Consequently, as air temperature warms, mulch begins to expand causing a waving effect to occur in the plastic. This can result is plastic mulch being blown off of the bed and/or injury to seedlings. Application of plastic mulch during warm conditions allows for a better stretch and fit of the mulch to the bed surface. The tighter the mulch fit the better is the bed warming effect and the earlier is the harvest time for the crop produced.
Polyethylene film, 0.9 to 1.5 mils thick by 4 feet wide is the most commonly used plastic mulch. The greatest use of plastic mulch has been with transplanted vegetables. Several commercially available transplanters do an excellent job of setting plants through the plastic mulches. Sources for transplanters can be found in Appendix, Table 18. Additionally, several plug mix planters and seeders have the capability of direct seeding vegetables through plastic mulches. Because of the cost associated with the use of plastic mulch, double cropping of the mulched area is becoming a common practice.
As with most precision farming equipment, mulch applicators will function properly only on well prepared seed beds. Slightly raised beds with well pulverized soils are most desirable. These beds allow for a tight stretch of the plastic, good soil to plastic contact which is conducive to uniform bed warming and uniform moisture distribution, and easy covering of plastic edges which securely anchors the plastic. If good coverage is not achieved, plastics will be blown out of place by wind gust. The use of windbreaks in combination with plastic mulches reduces the incidences of plastic blowing out. Additionally, clod free soils are a must to prevent mulch tearing while be stretched over the bed surfaces by the applicators.
Row Covers
Row covers have been used for many years to favorably modify climates and enhance early growth and yield of certain vegetables. New materials and methods developed recently make row covers a viable production practice wherever vegetables are seeded or transplanted when temperatures are below optimum and early production is desired. Row covers, when properly used, will result in earlier harvest and perhaps greater total production. There are two general types of row covers, supported and floating. Many variations of the row cover concept are possible, depending on the needs of individual growers. Row covers work best when used in conjunction with black polyethylene mulched rows or beds. Pepper, cabbage and onion transplants have been successfully grown under row covers on the Texas High Plains.
Supported Row Covers
Clear polyethylene, 5 to 6 feet wide and 1 to 1.5 mils thick, is the most convenient material to use and is generally used just once. Slitted row covers have slits, 5 inches long and ¾ inches apart, in two rows. The slits, arranged at the upper sides of the supported row cover to provide ventilation; otherwise the cover would have to be manually opened and closed each day to prevent plant injury or death from heat buildup. Hoops of No. 8 or No. 9 gauge wire are cut 63 inches long for use to support 5 feet wide polyethylene. Hoops are installed over the crop so that the center of the hoop is 14 to 16 inches above the row. Slitted row cover can be mechanically applied over the hoops with a high clearance tractor and a modified mulch applicator.
Floating Row Covers
Floating row covers are made of spun bonded polyester and polypropylene which is similar to fabrics used in the clothing industry for interlining and interfacing. It is white or off white, porous to air and water, very lightweight (0.6 oz/sq. yd) and transmits about 80% of the light. The material comes in rolls 67 inch wide and 250 to 2,500 feet long. One piece blankets are also available. With care, the spun bonded fabrics can be used two to three or more times.
Immediately after planting (seeds or transplants) the spun bonded fabric is laid directly over the row, and the edges are secured with soil, boards, bricks, or wire pins. Because the material is light weight, the plants support the fabric on their leaves as they grow. Enough slack should be provided to allow for the plants to reach maximum size during the time the material is left over them. For pepper or tomato, about 12 inches of slack should be left. For a crop such as cantaloupe, 8 inches is sufficient.
Floating covers can be left over vegetables for 3 to 8 weeks, depending on the crop and the weather. For tomato and pepper it can be left on for about 1 month but should be removed (at least partially) when the temperature under the covers reaches 86F and is likely to remain that high for several hours.
Muskmelon blossoms can withstand very high temperatures, but the cover must be removed when the first female flowers appear enable bee pollination.
Frost Protection
Frost protection achieved with slitted and floating covers is not as good as with solid plastic covers. A maximum of 3 to 4 degrees F is all that can be expected, whereas with solid covers, frost protection of 5 to 7 degrees F has been attained. Row covers should not be viewed only as a frost protection system, but as a growth intensifying system during cool spring weather. Therefore, do not attempt to plant early and hope to be protected against heavy frosts. An earlier planting date of 10 days to 2 weeks would be more reasonable. The purpose of row covers is to increase productivity through an economical increase of early and perhaps total production. Harvest of fruiting vegetables can be advanced four to six weeks by early transplanting under row covers as compared to seeding as usual.
Covered Trench
Covered trench (depression) is another method to modify the seed bed microclimate to enhance earliness in certain vegetable crops. This technique is a variation of the Rainfall Capture technique described above. Seed beds are configured in the same manner. However, with this method seeds are sown in the bottom of the trench or depression in the bed surface. Perforated clear plastic mulch is applied over the trenched. The perforations should be in a pattern as to line up over the trenched areas. Unlike with the system for RFC, the plastic mulch is not made to conform to the shape of the trench. Slitted row covers, described for use as supported row covers, work well for this concept. Once seedling growth has progressed to the point where the vegetation comes in contact with the plastic, the mulch is removed. This is also effective as a partial RFC technique as well as one that affords frost protection to early seedling growth.
Crop Training
Crop training can alleviate crop stress of some crops. Vining vegetables such as bean, tomato, and cucumber prostrate stems along the bed surface. When fruits of these plants that contact the soil surface they are prone to attack from soil borne pathogens. Vining plants foliage stay wet longer when exposed to dews, rainfall and/or irrigation. Foliage diseases are more of a problem. Similarly, weed control with cultivation can be difficult if herbicides are not used or fail to give control. By training vines of these crops to grow upward off of the bed surface, these and other problems can be managed more effectively. Tomato, pole bean and cucumber are the most commonly trained vegetables.
Staking
Staking and pruning was the earliest method of training tomatoes. With this method, all suckers (small shoots which grow out of the point of leaf attached to the stem) are removed with the possible exception of one or two. Normally only one central vine is allowed to develop from which 4 to 5 clusters of fruit are harvested. Shortly after field setting, a stake approximately 6 feet in length is driven into the soil near the plant (within 3 to 4 inches). The plant is then loosely tied to the stake. Tying is generally repeated 3 to 4 times. The major advantage of staking is an early concentration of large fruit. However, total fruit yield is usually reduced (See Figure IV-5).
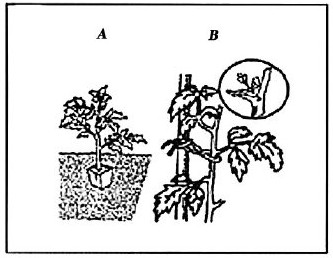
Figure IV-5. Staking, tying and pruning tomatoes.
Trellising
Training vines to grow up a trellis is another effective means of forcing vegetable plants to grow vertically. The trellis can be of a permanent type similar to that used in grape culture or a temporary type (Figures IV-6 and IV-7). Trellised tomatoes are not pruned when determinate hybrids are grown. There are many types of temporary trellises but the woven strip trellis is the least expensive. With this method, well anchored posts are at 15 feet intervals within the plant row and two smaller stakes are driven into the soil at 5 foot intervals between posts. Shortly after field setting the transplants, two heavy duty strings are woven on opposite sides of each plant from post to stake to post. This procedure is repeated with each additional foot of new growth. Training to trellises is also appropriate for indeterminate growing beans and cucumbers. In addition to reducing diseases and aiding cultivation, harvest efficiency is greatly improved by crop training techniques.
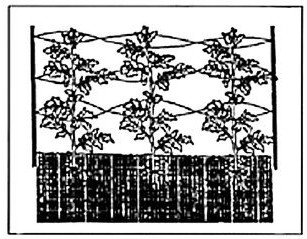
Figure IV-6. Woven strings trellising of tomatoes.
Caging
Caging is another training technique well adapted to tomato cultures. As the name implies, tomato plants are allowed to grow upward within wire cages. Wire cages are easily constructed from pieces of 10-gauge concrete reinforcement wire having a 6″ x 6″ mesh and 5 ft in height and 6 ft in width. Each piece is then hooked together forming a cylinder 15 to 18 inches in diameter. These cages are placed over the plants before they start to vine or soon after transplanting (Figure IV-8). The plants are then allowed to develop normally.
These techniques perform better when they are used in conjunction with black plastic mulch. All of these training techniques can also be covered with cloth type row covers or clear ‘Vispore’ plastic materials for the added advantage of frost protection.
Recently, individual cage covers made of these products have been introduced. Such covers seem to provide at least temporary control to certain insect problems. As with all row covers they should be removed once the threat of frost has past, or just prior to the onset of consistently high air temperature or when plant flowers must be made accessible to bees.
Use of Honeybees
Fruit formation and seed development in vegetable plants depends on a pollination and fertilization. Inadequate pollination results in poorly developed, misshaped, unsalable fruit. Weather extremes can cause flowers to drop and poor fruit set can in spite of good pollination. In most cases, pollination involves the transfer of pollen from male structures (anthers) of the flower to the female structures (pistils) of flowers. Pollen is also used as a food source by a wide spectrum of insects. It is especially important to a number of bee species, whose bodies and life habits are well adapted to the activity of pollen collection. In the process of collecting this valuable food source, these insects provide the pollen transportation link that many plants need for reproduction. A single honeybee can carry up to five million pollen grains on its body, and a strong honeybee colony may bring in well over 50 pounds of pollen during a season.
Other factors influence the honeybee’s role as the pollinating leader. Only honeybees are significantly socially developed to overwinter and to perpetuate their colonial life. This is the key element which makes management of honeybees possible. Populations of naturally occurring bee species have been declining for the last few decades. This may be due to a number of factors including the reduction of natural habitats, an increase in pesticide use, and fewer rural living people engaged in bee keeping.
At least 90 crops grown in the United States depend to some extent upon bees as pollinators, either for seed or for fruit production. Listed below are a number of important crops that must be insect pollinated to produce fruit and the number of hives suggested to achieve adequate pollination. The exact number of hives needed will depend on a number of factors including strength and condition of colonies, magnitude of the natural pollinator community, and amount of wild flower material competing with the crop, attractiveness of the crop to bees, projected yield, and the weather. The following are guidelines for the number of hives to use when supplemental pollination is desired:
- Cucumber: at least 1 colony per acre
- Squash: 1 to 3 colonies per acre
- Muskmelon: 1 to 3 colonies per acre
- Watermelon: 1 colony per acre
- Pumpkin: 1 to 3 colonies per acre
The following vegetables will set fruit without bees, but bee activity has shown to increase yields: eggplant, lima bean, okra, pepper, and greenhouse tomato. Conversely, honeybees do not assist in the pollination of the following crops, but will collect pollen and nectar from them: peas, snap bean, sweet corn, and tomato.
Many growers currently purchase pollination services from beekeepers for their vegetable fields. To insure mutual satisfaction of both the grower and the beekeeper, a pollination contract should be signed by both. The following points should be considered in the contract: rental price, number of colonies, strength of colonies, timing of placement in the fields and removal from fields, field location, responsibility for maintenance of the colonies, access for the beekeeper to the hives, liability for stings, and protection from pesticides.
Generally, a grower should require that any hive rented for pollination should be disease free and have two deep supers (boxes); a good egg laying queen; at least of 4 to 5 frames of immature bees; and 20 pounds of surplus honey. Hives should only be placed in fields after the crop’s flowers are available to visit by the bees. If hives are placed before there are available flowers, bees will forage in other surrounding areas. Once an individual bee begins foraging a particular type of flower, it tends to stick with that flower and may not pollinate the intended field when flowers become available. Also, in planning the placement of hives, growers should remember that honeybees usually pollinate flowers most thoroughly within 100 yards of their colony, although if forced to, they will travel much farther. Thus, hives should be distributed in groups throughout the field rather than in a single location, as a convenience to the beekeeper and to aid uniform pollination.
Certain pesticides and pesticide application practices pose a serious hazard to honeybees. In general, night applications or later afternoon applications pose the least hazard to bees. The application of a single pesticide is usually less dangerous than applying combinations. Systemic insecticides are usually very safe to bees, as well as granular formulations. Emulsifiable or water soluble formulations are safer than wettable powders of the same material. Insecticides of varying degrees of toxicity to honeybees (listed in descending order of toxicity) are presented in Table IV-3.
Table IV-3. Degree of Toxicity of Pesticides on Honeybees
Highly Toxic: Severe bee losses can be expected if the following materials are used when honeybees are present at treatment time or within a day thereafter. |
Moderately Toxic: These can be used in the vicinity of honeybees if the dosage, timing, and method of application are correct. However, they should not be applied directly on honey bees in the field or at the hives. |
Relatively Non-Toxic: This group of materials can be used with a minimum of injury. |
---|---|---|
chlorpyrifos (Lorsban) | disulfoton (Di-Syston) | Bacillus thuringiensis (Dipel) |
carbofuran (Furadan) | endosulfan (Thiodan) | dicofol (Kelthane Miticide) |
Parathion | phorate (Thimet) | Pyrethrum |
dimethoate (dimethoate) | oxamyl (Vydate) | Rotenone |
methidathion (Supracide) | Trichlorfon | |
methyl parathion (Penncap-M) | ||
diazinon (Diazinon) | ||
azinphosmethyl (Guthion) | ||
naled (Dibrom) | ||
malathion (Cythion, Malathion) | ||
phosmet (Imidan) | ||
acephate (Orthene) | ||
carbaryl (Sevin) | ||
methamidophos (Monitor) |
Adapted from the Indiana Vegetable Production Guide for Commercial Growers.