V. A. Haby, Marvin L. Baker, and Sam Feagley
Soil is a habitat for plants. As such, the soil’s physical, chemical, and biological properties affect plant growth. The physical properties of a soil largely determine the ways in which it can be used. The size, shape, and arrangement of the primary soil particles are known as the physical properties of soil. Other important physical properties center on these such as the size and shape of the spaces between the particle arrangements, called the pore space, which has a direct effect on the movement of air and water, the ability of the soil to supply nutrients to plants, and the amount of water available to the plant.
The proportions of the four major components of soils, inorganic particles, organic materials, water, and air, can vary greatly from place to place and with depth. The amount of water and air in a soil can also fluctuate widely from season to season. However, the physical characteristics of the solid components, inorganic and organic particles, are essentially unchanging.
Chemical properties of soils are important in that, along with their physical and biological properties, they regulate the nutrient supplies to the plant. Without these nutrients supplied by the soil or applied as inorganic fertilizers, organically by manures, and other vegetative materials, plant growth would cease.
The biological properties of the soil are dictated by the macroorganisms and microorganisms. Good physical and chemical properties supply the right environment and sufficient nutrients to the organisms for optimal biological activity. This in turn improves the soil physical and chemical properties through improved structure and nutrient cycling.
Important Definitions and Considerations
Soil Structure: The arrangement of the primary soil particles into clusters or masses called aggregates.
Primary Soil Particle: Mineral particles sizes including sand (0.05 to 2 mm), silt (0.002 to 0.05 mm), and clay (<0.002 mm.)
Soil Porosity: The ratio of the volume that is not occupied by soil particles to the bulk volume of the soil is called soil porosity. It is the degree to which the soil mass is permeated with pores or cavities.
Pore Space: The solid soil particles (minerals and organic matter) occupy about 50% of the total volume in a soil. The remaining space, the voids between the solid particles and between the aggregates, is called the pore space. The pore space is the volume occupied by water and the soil air.
Soil Air: Water and air occupy the soil pores in varying amounts. The amount and composition of the air in a soil have important direct and indirect effects on vegetable growth.
Soil air differs from the atmosphere above the soil in that it usually contains 10 to 100 times as much carbon dioxide, slightly less oxygen, and is saturated with water vapor.
Soil Air Movement: Air enters and leaves the soil through cracks in the soil and through the pores at the surface of the soil.
Saturation of the pores with water limits the movement of oxygen into and carbon dioxide out of the soil. This condition can occur from over-watering or from poor drainage of excess water down through the soil profile. A waterlogged condition can severely damage or kill the roots of plants. It is not the water that inflicts the injury, but the lack of oxygen. Therefore, drainage of excess water from a soil is important to vegetable growth.
Soil Water: Water moves through the pore spaces of a soil. The pore spaces are also where water is retained for plant use.
Sandy soils tend to have a preponderance of large pores whereas clayey soils are much smaller. During and following the entry of rain and irrigation water, sandy soils with their larger pores, usually conduct water more rapidly than clayey soils. For that reason, sandy soils retain less water for plant use. The loam textures have the highest plant available water.
Drainage: The rate at which water moves from the root zone is known as drainage. If the soil is kept excessively wet for long periods of time, oxygen cannot reach the roots and damage can occur. Sometimes a soil layer exists in the profile that restricts the downward movement of water. In these cases drainage is slowed or stopped because a water table forms above the restricting layer.
Soil Organic Matter: Organic matter is a temporary product or a stage in a natural cycle of decay or decomposition of plant and animal residue. Soil organic matter is dynamic. It changes continually through further decomposition. The amount of organic matter affects the physical properties of soil. Organic matter acts as biological “glue” that cements individual soil particles together into aggregates. Thus, it affects soil structure and the retention and movement of water and air into, in and out of the soil. Dead plant matter provides food for microorganisms that in turn die and make a contribution to the total organic content of the soil. Some organisms such as earthworms while feeding on organic matter modify the structure of the soil and improve its porosity.
Soil Temperature: The temperature of soils varies at different depths and seasons of the year. The changes are determined by the amount of the radiant energy that reaches the soil surface and by the thermal properties of the soil. Dark soils absorb a much higher proportion of radiant energy than do light colored soils. Energy that has been absorbed is disposed of in one or more of the following ways by reradiation to the atmosphere; by heating of the air above the soil by convection; by increasing the temperature of the surface soil; or by conduction to the deeper soil layers.
Soil Crusts: Soil crusts are formed by the desiccation of a thin layer of dispersed surface soil. Such crusts frequently have considerable mechanical strength and may keep vegetable seedlings from emerging or cause injury to the stems of established plants.
Soils and Plant Roots
Of equal importance are the quantitative aspects of the soil as a habitat for plant roots. It is necessary that nutrients, air, and water are present in optimum concentrations for normal root development and plant growth. It is also necessary that enough of them be present throughout the growing season to meet plant needs.
The depth of soil available for rooting is a major physical factor that influences vegetable growth. Severe limitations are placed on the ways in which the soil can be used when the rooting volume is restricted by bedrock, cemented layers, a high water table, or other root barriers.
The effective depth of a soil is determined by the total thickness of the soil layers that can be readily penetrated by plant roots. The roots of most vegetable crops will penetrate to a depth of 3 feet or more if soil conditions permit. A desirable soil should be favorable for the reception, transmission, and storage of water to a depth of at least 42 inches. The ability of a soil to withstand drought is related to its depth and chemical composition. Plant nutrients stored in the subsoil are used by plants if roots can reach them.
Vegetable Nutrition
There are 20 essential chemical elements (plant nutrients) known to be required for normal vegetable growth. These elements can be supplied by either organic or commercial inorganic fertilizers. Carbon, hydrogen, and oxygen from air and water, and nitrogen from organic and inorganic sources are four plant nutrients which make up 95% of plant solids. Although he atmosphere consist of 78% nitrogen as N2, this form is unavailable for plant use. However, certain bacteria that live symbiotically in nodules on the roots of legumes are able to take nitrogen from the air and fix it in a form available to plants.
The other 16 essential elements, iron, calcium, phosphorus, potassium, copper, sulfur, magnesium, manganese, zinc, boron, chlorine, molybdenum, cobalt, sodium, silicon and vanadium, are supplied to plants by the soil. With the exception of nitrogen and phosphorus, most alkaline, clay type soils usually contain enough of these elements for vegetable production. In acid sandy soils, nitrogen, phosphorus, potassium, calcium, magnesium and, at times, sulfur and boron may need to be added for successful crop production. Cobalt, sodium, silicon, and vanadium have been proven essential for only a few plants.
Soil pH
Soil pH indicates the hydrogen ion activity of soil solution or growth media. The number expresses the degree of acidity or alkalinity in terms similar to the way heat and cold are expressed in degrees of Centigrade or Fahrenheit. The scale for measuring acidity or alkalinity contains 14 levels known as pH units (Figure III-1). It is centered on pH 7 which is neutral. Values below 7.0 are acidic and values above 7.0 are alkaline. The pH scale is logarithmic, not linear. That is, a soil with a pH of 8.0 is ten times more alkaline than a soil with a pH of 7.0. A soil with a pH of 5.0 is 100 times more acid than a soil with a pH of 7.0.
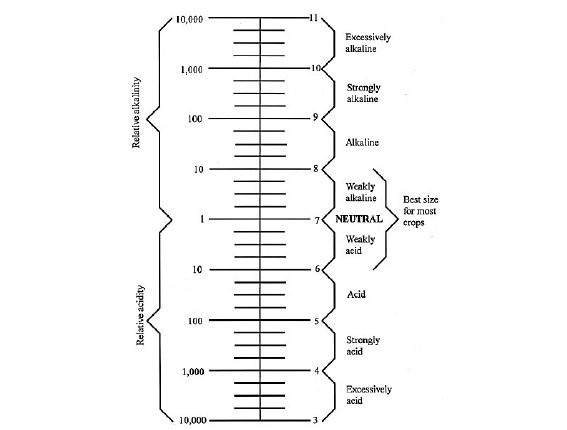
Figure III-1. Relation between pH, Alkalinity, Acidity, and Plant Growth
Soil pH is one of a number of environmental conditions that affect the quality of plant growth. A slightly acid soil is desirable for most vegetables. Some types of plant growth can occur anywhere in a pH range of 3.5 to 10.0. With some exceptions, a soil pH of from 6.0 to 7.0 is ideal for good plant growth. The major impact of pH extremes on plant growth is related to the availability of plant nutrients and the soil concentration of plant toxic elements. In strongly acid soils, pH < 5.5, manganese and aluminum can concentrate at toxic levels. Also at low pH values, calcium, phosphorus, magnesium, and molybdenum are less available. At pH values of 7.0 and above, phosphorus, iron, copper, zinc, boron, and manganese decrease in availability.
Adjustments can be made in pH values by the application of amendments to the soil. Finely ground agricultural limestone most frequently is used to decrease soil acidity and increase pH. The finer the grind, the more rapidly limestone neutralizes acidity. Different soils will require differing amounts of lime to adjust the soil pH to the proper range, usually 6.5 to 7.0. The texture of the soil, organic matter content, and the crop to be grown are factors to consider in adjusting pH. For example, low organic matter soils require less lime to make the same pH change than soils high in organic matter.
If pH is too high, elemental sulfur, concentrated sulfuric acid or aluminum sulfate can be added to the soil to decrease alkalinity. Caution should be taken when adding these acidifying materials. If they are over applied, the pH can drop into the 2 to 3 values in small areas of the soil and potentially kill plants in these areas. Iron chlorosis, a deficiency of iron in the plant, can be corrected by applying chelated iron or iron sulfate to the soil or foliage. The term chelate comes from the Greek word claw. Chelates are chemical bonds (claws) that help hold metal ions, such as iron, in solution so that plants can absorb them. Different chemicals, from relatively simple natural chelates like citrate, to more complex manufactured chemicals, can act as chelates. When a chelated metal is added to the soil, the nutrient held by the chelate will remain available to the plant longer than if the sulfate salt is applied to the soil. Most nutrients do not require the addition of a chelate to help absorption. Only a few of the metals, such as iron and zinc, benefit from the addition of chelates. The types of chelate used will depend on the nutrient needed and the soil pH. Iron is normally applied foliarly, just wetting the leaf, using a 2% iron solution. This is because the soil binds with the iron so rapidly that as much as 10 to 15 lb of iron may not meet the plant iron requirement. Zinc can be applied foliarly using a 0.5% zinc solution or applied to the soil. The chelated form works best for soil application. The sulfate or chelated form can be used for foliar application.
Fertilizer Grade or Composition
Fertilizer bags are labeled with at least three numbers. These numbers list the percentage of nitrogen (N), available phosphate (as P2O5) and soluble potash (as K2O). These numbers represent nitrogen, phosphorous and potassium, commonly referred to as N-P-K. However, these elements are symbolically represented as N-P2O5-K2O. For example, if we have a 100 pound bag of fertilizer labeled 10-10-10, it contains 10 pounds of N, 10 pounds of P2O5, and 10 pounds of K2O. To convert to actual phosphorus, multiply the P2O5 by 0.44 and, to convert to actual potassium, multiply the K2O by 0.83. The other 70 pounds is filler or carrier which is important to help spread the fertilizer evenly and avoid burning plants with too much fertilizer. A 100 pound bag of fertilizer labeled 0-20-10 would contain no N, 20 pounds of P2O5, 10 pounds of K2O and 70 pounds of filler or carrier. Fillers may be clay, saw dust etc. Other parts of the nutrient carrier may be other elements associated with the nitrogen, phosphorous, and potassium such as hydrogen, oxygen, calcium, and chloride.
Many states have adopted a model label law for the classification of fertilizers. The law establishes minimum levels of nutrients allowable and provides specific labeling requirements. To date, model label legislation has not met with total acceptance, so there are still differences from state to state as to what constitutes a fertilizer, and the type of information required on labels. Even so, the information contained on fertilizer labels has been well standardized. The consumer is protected by state laws requiring manufacturers to guarantee the nutrients claimed percentages. Samples are periodically collected from fertilizer lots and analyzed by the Feed and Fertilizer Control Service at College Station to ensure the guaranteed amounts of each nutrient. The law requires only that the manufacturer guarantee what is claimed on the label. In some cases, a fertilizer will contain secondary nutrients or micronutrients not listed on the label because the manufacturer does not want to guarantee their exact amounts.
Some mention should be made of the initials or designations W.I.N. and W.S.N. on fertilizer labels. These stand for water insoluble nitrogen and water soluble nitrogen, respectively. The W.S.N. dissolves readily, and is usually in very simple form such as ammonium or nitrate nitrogen. Nitrogen which will not dissolve readily may exist in other forms in the fertilizer and is called W.I.N. These usually are organic forms of nitrogen (with the exception of urea) that must be converted to simpler forms before they can be used. Water insoluble nitrogen (W.I.N.) is referred to as a slow release nitrogen source which delivers nitrogen at different rates according to the amount and kind of material of which it is composed.
The best fertilizers to use for growing vegetables depend on many factors, such as which nutrients are needed, the soil structure and chemistry and the method that will be used to apply the fertilizer. The best method of estimating which and how much nutrients are needed is through soil testing and recommendations based on crop and yield goals.
Complete vs. Incomplete Fertilizers
A fertilizer is said to be complete when it contains the major nutrients, nitrogen, phosphorus and potassium. The manufacturers of commercial fertilizers are required to state the analysis (amounts of these nutrients) on the container and guarantee the analysis. Examples of commonly used fertilizers are 13-13-13, 16-6-12 and 10-20-10. An incomplete fertilizer will be missing one of the major nutrients. Examples of incomplete fertilizers are indicated in Table III-1.
Table III-1. Common Incomplete Fertilizers or Farm-Type Fertilizers
Fertilizer | %N | %P2O5 | %K2O |
---|---|---|---|
Ammonium Nitrate | 34 | 0 | 0 |
Ammonium Sulfate | 21 | 0 | 0 |
Monoammonium Phosphate | 11 | 48 | 0 |
Muriate of Potash (potassium chloride) | 0 | 0 | 60 |
Potassium Sulfate | 0 | 0 | 52 |
Super Phosphate | 0 | 20 | 0 |
Triple Super Phosphate | 0 | 45 | 0 |
Urea | 46 | 0 | 0 |
Urea-ammonium nitrate (liquid) | 32 | 0 | 0 |
Complete fertilizers can be made by blending incomplete fertilizers. For example, if 100 pounds of urea (46-0-0) were combined with 100 pounds of triple super phosphate (0-45-0) and 100 pounds of muriate of potash (0-0-60), 300 pounds of a fertilizer grade of 15-15-20 would result. This tells us that 100 pounds of urea fertilizer originally contains 46 pounds of nitrogen; triple super phosphate, 45 pounds of phosphate; and, muriate of potash, 60 pounds of potash. When these three quantities are combined, each original quantity is diluted two-thirds by the other two, and the result is 300 pounds of 15-15-20. The specific fertilizer ratio you will need depends on the soil nutrient level.
Special Purpose Fertilizers
A soil test should be performed before the purchase of any “special purpose” fertilizers. It is not possible to make a blanket statement that one fertilizer is best for vegetables in every area of the state. It is true that different vegetables use different nutrients at different rates. The unknown is the reserve of nutrients already in your soil. This reserve changes with every soil type, location, and previous fertilizer history of the soil.
Slow Release Fertilizers
Vegetable plants take up nutrients continuously. It is beneficial to provide them with a balance of nutrients throughout their growth. At times, the most efficient way to achieve this is to apply slow release fertilizers which are designed to release nutrients over a relatively long period of time. Slow release fertilizers contain one or more plant essential elements. They can be categorized by the way in which these elements are released. The major types of nutrients are released from materials that: dissolve slowly; from which the nitrogen is released by microorganisms; and, from granular materials with membranes made of resin or sulfur that controls the rate of nutrient release from the granules into the soil.
Sulfur-coated urea is a slow release fertilizer with a covering of sulfur around each urea particle. Different thicknesses of sulfur control the rate of release of nitrogen. Increasing temperature increases the nitrogen release rate while watering does not affect it. Sulfur-coated urea applied to the soil surface releases more slowly than it does if it is incorporated into the soil. This material generally costs less than other slow release fertilizers, and it supplies the essential element, sulfur.
When fertilizer products coated with multiple layers of resin contact water, the layers swell and increase the pore size in the resin so that the dissolved fertilizer can move into the soil. The release rate depends on the coating thickness, temperature, and water content of the soil. There is often a significant release of fertilizer during the first 2 or 3 days after application. Release timing can range from 0 to 6 months depending on the coating. With high temperatures experienced in Texas, high release rates and salt damage are frequently observed. Slow release fertilizers need not be applied as frequently as other fertilizers, and higher amounts can be applied without danger of burning. Vegetable plants may use the nitrogen in slow release fertilizers more efficiently than in other forms because it is being released continually over a longer period of time than in conventional fertilizers. Slow release fertilizers generally are more expensive than other types. The real benefit is the reduced frequency of application for slow release materials. Advantages and disadvantages of conventional and slow release fertilizers are listed in Table III-2.
Table III-2. Comparison of Conventional and Slow Release Fertilizers
Type of Fertilizer | Advantages | Disadvantages |
---|---|---|
Slow Release Fertilizer |
|
|
Conventional Fertilizer |
|
|
Manures or Sewage Sludge |
|
|
The word organic applied to fertilizers simply means that the nutrients contained in the product are derived solely from the remains or a byproduct of a once living organism. Urea is a synthetic organic fertilizer, an organic substance manufactured from inorganic materials. Cottonseed meal, blood meal, bone meal, hoof and horn meal, and all manures, are examples of organic fertilizers. Most of these products packaged as fertilizers will have the nutrient ratios stated on the package labels. Some organic materials, particularly composted manures, and sludge, are sold as soil conditioners. Although nutrients are present in small amounts, these products do not have a nutrient guarantee. Most contain one or more of the three major nutrients in low concentrations, although you may find some fortified with nitrogen, phosphorous or potassium for a higher analysis. In general, organic fertilizers release nutrients over a fairly long period; the potential drawback is that they may not release enough of their principal nutrient at a time to give the plant what it needs for best growth. Because organic fertilizers depend on soil organisms to mineralize and release nutrients, most of them are effective only when soil is moist and soil temperature is sufficiently warm for the soil organisms to be active.
Cottonseed meal is a byproduct of cotton production. As a fertilizer, it is somewhat acid in reaction. Formulas vary slightly, but generally contain 7% nitrogen, 3% phosphorous, and 2% potassium (Table 19 in Appendix). Nutrients in cottonseed meal are more readily available to plants in warm soils, but there is little danger of burn. Blood meal is dried, powdered blood collected from cattle slaughterhouses. It is a rich source of nitrogen, so rich, in fact, that is may do harm if used in excess. The vegetable producer must be careful not to exceed the recommended amount suggested on the label. In addition to nitrogen, blood meal supplies some essential trace elements, including iron, as a well rounded fertilizer.
Fish emulsion, a well rounded fertilizer, is a partially decomposed blend of finely pulverized byproducts form processing fish for human consumption. No matter how little it is used, the odor is intense, but it dissipates within a day or two. Fish emulsion is high in nitrogen, and is a source of several trace elements.
Manure varies in nutrient content according to the animal source and its diet but a fertilizer ratio of 1-1-1 (as N2-P2O5-K2O) is typical. Manures are best used as soil conditioners instead of nutrient suppliers. Horse, cow, pig, chicken, and sheep manures are commonly available. The actual nutrient content varies widely. The highest concentration of nutrients is found when manures are fresh. As it is aged, leached or composted, nutrient content is changed.
Even though fresh manures have the highest amount of nutrients, vegetable producers should use composted forms of manure to ensure a lesser amount of salts, thereby reducing the chance of burning plants. Fresh manure should not be used where it will contact tender plant roots. Typical rates of manure application vary from a low 70 pounds per 1,000 sq. ft (1.5 T/A) to a moderate level of 185 pounds per 1,000 sq. ft (4.0 T/A). Rates over 345 pounds per 1,000 sq. ft (7.5 T/A) should be considered excessive for most manures. Cow manure can be applied up to 925 pounds per 1000 sq. ft (20 T/A). These rates should not be applied but once every two years. These rates are based upon dry weight.
Sewage sludge is a recycled product of municipal sewage treatment plants. Two forms are commonly available, activated and composted. Activated sludge has higher concentrations of nutrients (approximately 6-3-0.2 as N-P2O5-K2O) than composted sludge, and usually is sold in dry, granular form for use as a general purpose, long lasting, non burning fertilizer. Composted sludge, is used primarily as a soil amendment, and has a lower nutrient content (approximately 1-2-0.2 as N-P2O5-K2O). There is some question about the long term effect of using sewage sludge products. Heavy metals such as cadmium are some items present in the sludge, and may concentrate in the soil and plants. Possible negative effects vary, not only with the origin of the sludge, but also with the characteristics of the soil and where it is used. You are encouraged to request an analysis of heavy metal content of the sludge before purchasing it. If the sludge is labeled as class A, then the metals will not be a concern.
Compared to synthetic fertilizer formulations, organic fertilizers contain relatively low concentrations of actual nutrients, but they perform other important functions which the synthetic formulations do not. These functions include increasing organic matter content of the soil, improving physical structure of the soil and increasing bacterial and fungal activity, particularly the mycorrhizal fungi which alone make other nutrients more available to plants. Table III-3 shows the approximate nutrient content of manures, and suggested rates of annual application. Again it is best to apply manures and other organic fertilizers at soil test recommended rates, rather than suggested yearly rates.
Fertilizer Formulations
Different formulations of fertilizers are made to meet the needs of different situations. All formulations must give the amount of nutrients, and it may tell how quickly a nutrient is available. Some formulations available to the homeowner include water soluble powders, slow release pellets, liquids, and granular materials.
Table III-3. Approximate Nutrient Content of Manures and Suggested Yearly Rates of Application per 1,000 Square Feet of Area
Manure Type(dry) | % N | % P2O5 | % K2O | Suggested amounts of material (lb per 1000 sq. ft) |
---|---|---|---|---|
Chicken Manure | 2.0 – 4.5 | 4.6 – 6.0 | 1.2 – 2.4 | 125 |
Steer Manure | 0.6 – 2.5 | 0.9 – 1.6 | 2.4 – 3.6 | 450 |
Dairy Manure | 0.6 – 2.1 | 0.7 – 1.1 | 2.4 – 3.6 | 600 |
* Cut rates by 50% if soils are saline, or water has a medium to high salinity hazard.
Liquid fertilizer formulations include complete formulas and special types that offer just one or two nutrients. All must be diluted with water; some are concentrated liquids themselves, and others are powder or pellets.
Fertilizers will not burn or damage plants if they are applied correctly. Fertilizers are salts much like table salt, except that they contain various essential plant nutrients. When a fertilizer is applied to a soil, nearby water begins to move very gradually toward the area where the fertilizer has been applied. Fertilizer salts begin to diffuse, or move away from the place where they were applied. This dilutes the fertilizer and distributes it throughout a much larger area. If tender plant roots are close to the placement of a fertilizer, water is drawn from these roots, as well as from surrounding soil. The more salt or fertilizer applied, the more water is drawn from nearby roots. As water is drawn from the roots, plant cells begin to dehydrate and collapse and the plant roots burn or dehydrate to a point where they cannot recover. If soil moisture is limited, most of the water drawn toward the salt will come from the plant roots and the damage will be severe.
Two rules should be kept in mind when applying a fertilizer during hot weather and when soil moisture is limited:
- Do not over apply nitrogen fertilizers
- Make sure adequate moisture is present after applying fertilizers high in salts.
Table III-4 lists commonly used fertilizers and their salt content or burn potential.
Soluble salts build up when fertilizer is applied repeatedly without sufficient water to leach or wash the old fertilizer or salts through the soil. It also occurs when water evaporates from the soil and minerals or salts stay behind. As the salts in the soil become more concentrated, plants find it harder to take up water. If salts build up to an extremely high level, water will move out of adjacent root tips, causing them to die.
Fertilizer Application
Soil type dictates the frequency of fertilizer application. Sandy soils require more frequent applications and lower amounts of nitrogen and other nutrients than do clay type soils. Other factors affecting application frequency include the crop being grown and its intended use, the frequency and amount of nitrogen or water applied, and type of fertilizer used and its release rate. The crop influences timing and frequency of application because some crops are heavier feeders on some nutrients than others. Root crops require less nitrogen fertilization than leafy crops. Sweet corn is a heavy feeder of nitrogen, and may require nitrogen fertilization two or more times during its growth. A general rule of thumb is that nitrogen is for leafy top growth; phosphorus is for root and fruit production; and potassium is for cold hardiness, disease resistance, drought tolerance, and general durability. Proper use of nutrients can control plant growth, rate, and character. Nitrogen is the most critical nutrient in this regard. If tomatoes are fertilized too heavily with nitrogen, the plant may be all vines and no fruit. This is also the case with potatoes which will show excess vining and poor tuber formation. Slow release fertilizer or heavy amounts of manure used on crops that form fruit will maintain vegetative growth and fruit, or vegetable development will occur late in the season. Remember that a nitrogen application will have its greatest effect for 3 to 4 weeks after application. Table 22 of the Appendix list appropriate nutrient requirements of selected vegetables.
Application Methods
There are different methods of applying fertilizer depending on the formulation and the crop needs. These methods are discussed below.
Broadcast: A recommended rate of fertilizer is spread over the growing area and should be mechanically incorporated into the soil.
Band: Narrow bands of fertilizer are applied in furrows 2 to 3 inches to the side of the planting area and 1 to 2 inches deeper than the seeds or plants that are to be planted. Placement of the fertilizer band too close to the seeds can burn the roots of the seedlings depending on the fertilizer material banded (see Table III-4.)
Banding is one way to satisfy the phosphorus need of many vegetables as the first roots develop. When fertilizers are broadcast and worked into soil, much of the phosphorus is tied up by the soil and is not available to the plant. By concentrating phosphorus in a band, the plant is given what it needs and less of the phosphorus becomes tied up in the soil.
Table III-4. A List of Commonly Used Fertilizers and Salt Index or Burn Potential
Material | Analysis | Burn Potential per Equal Weights of Material |
---|---|---|
Ammonium Nitrate | 33% N | 104.7 |
Ammonium Sulfate | 21% N | 69.0 |
Potassium Nitrate | 14% N | 73.6 |
Urea | 46% N | 75.4 |
URAN | 32% N | 74.2 |
Concentrated Super Phosphate | 45% P2O5 | 10.1 |
Diammonium Phosphate | 21% N; 54% P2O5 | 34.2 |
Superphosphate | 20% P2O5 | 7.8 |
Potassium Chloride | 60% K2O | 116.3 |
Potassium Sulfate | 54% K2O | 46.1 |
Dolomite | 30% Calcium Oxide 20% Magnesium Oxide |
0.8 |
Gypsum | 33% Calcium Oxide | 8.1 |
Epsom Salt | 16% Magnesium Oxide | 44.0 |
Salt Index | Sodium Nitrate | 100 |
Starter Solutions: Another way to satisfy the need for fertility when setting out transplants of tomatoes, eggplant, peppers, etc. is through the use of a starter solution. Follow directions on the fertilizer label (refer to section on transplants in Chapter V).
Sidedress: Fertilizer may be applied as a side dressing after plants are growing. Fertilizer should be applied on both sides of the row 6 to 8 inches from the plants. Side dress soil incorporated applications should be made at a sufficiently young stage of growth in order to minimize root pruning.
Foliar Feed: This method is used when insufficient fertilizer was applied before planting; a quick growth response is wanted; micronutrients (such as iron or zinc) are locked into the soil; or when the soil is too cold for the plants to extract or use the fertilizer applied to the soil. Foliar applied nutrients are rapidly absorbed and used by the plant. Absorption begins within minutes after application, and with most nutrients, it is completed within 1 to 2 days. Foliar fertilization can be a supplement to soil fertilization at a critical time for the plant, but not a substitute. At transplanting time, an application of phosphorus spray will help in the establishment of the young plant in cold soils. Under cool soil conditions, microorganisms are not sufficiently active to convert nutrients into forms available for roots to absorb even if the nutrients were available, the plants could grow. A nutrient spray for foliage will provide the needed nutrients to the plants immediately, allowing them to begin growth. When using a foliar spray for secondary and trace nutrients add 1 teaspoon of a liquid dishwashing detergent per gallon of solution or add a commercial surfactant at the recommended rate to break the surface tension of the solution and prevent beading on the foliage. Apply only the spray volume needed to wet the foliage without dripping or running liquid off the leaves.
Soil Testing
Soil tests for vegetable production involve the evaluation of plant nutrient availability in soils. Knowledge of the nutrient availability status allows an interpretation to be made for applying only those nutrients not already adequate in the soil. Generally, the regular or standard soil analysis for pH, nitrogen, phosphorus, potassium, calcium, magnesium, sodium, sulfur and salinity should be adequate for most garden fertility needs. However, tests also are available for the micro nutrients zinc, iron, copper, manganese and boron. When a deficiency of one of these is suspected, request that the soil be analyzed for that nutrient.
Test results will be interpreted by the laboratory and fertilizer suggestions will be provided. If needed, a suggested rate of limestone to apply will be provided for acid soils. Soil sample bags and information sheets are available from your county Extension office where assistance also can be obtained with questions regarding the test results and fertilizer suggestions. The procedure for collecting the sample and mailing it to the laboratory is described on the backside of the information form. The information provided in Table 23 of the Appendix can be helpful in interpreting test results. If soil pH is sufficiently low to warrant liming, as is the case in many places in East Texas, appendix Table 30 shows the quality of lime required per acre to correct a low pH problem and presents the type of lime and their neutralizing equivalents.
Normally pH can be lowered by the addition of sulfur to the soil (Table 31 of Appendix). However, in the alkaline soils found in most areas of South, West and/or North Texas, lowering of the pH is very difficult. Irrigation in these areas creates a neutralizing affect due to the high CaCO3 levels normally present in the water.
Soil salinity is an important consideration in crop production. As soil salinity increases water extraction by plants from the soil solution is lessened. This situation is more critical under hot and dry conditions. Crop response can be severely affected by high soil salinity. Table IV-4 indicates crop response to salinity.
Table III-5. Crop Response to Salinity
Salinity (expressed as ECCE, mmho/cm, or dS/m) |
Crop Response |
---|---|
0 – 2 | Salinity effects mostly negligible |
2 – 4 | Yields of very sensitive crops may be restricted |
4 – 8 | Yields of many crops restricted |
8 – 16 | Only tolerant crops yield satisfactorily |
> 16 | Only a few very tolerant crops yield satisfactorily |
Plant Nutrient Deficiency Symptoms
Plants which become excessively deficient in one or more nutrients will display those deficiencies with distinctive discoloration patterns on the leaves and/or by characteristic leaf and stem distortions. However, even before these deficiency symptoms become visible, the plant can be suffering from a nutrient deficiency which is sufficiently severe to cause yield reduction. Soil testing and plant tissue analysis are two common techniques which may be used to prevent nutrient deficiencies in plants. Plant nutrients may be classed as mobile or immobile relative to the plant’s reaction to nutrient deficiencies. Nitrogen, phosphorus, potassium and magnesium are considered mobile nutrients. When the soil becomes deficient in these nutrients, actively growing plants will remove them from the older leaves and mobilize them to the young, actively growing leaves. Therefore, deficiencies of these four nutrients are first observed in the older leaves.
All other nutrients are classified as immobile because they are not readily resolubilized from the older leaves and moved to the new growth. Deficiencies of these nutrients will first appear in the new growth.
From this information, if the deficiency is showing on older leaves, compare it with the symptoms described for nitrogen, phosphorus, potassium and magnesium. Deficiencies showing on new growth will be from any of the other secondary and micronutrients. Table 20 of the Appendix describes the various nutrient deficiencies.
The authors would like to acknowledge Dr. Dale Pennington, Soil Scientist Retired, Texas AgriLife Extension Service for his contributions.