The process of decomposition — the breakdown of raw organic materials to a finished compost — is a gradual complex process, one in which both chemical and biological processes must occur in order for organic matter to change into compost.
The decomposition (stabilization) of organic matter by biological action has been taking place in nature since life first appeared on our planet. In recent times, man has attempted to control and directly utilize the process for sanitary recycling and reclamation of organic waste material. Such organic materials as vegetable matter, animal manure and other organic refuse can be converted from otherwise wasted materials to a more stable form for use as a soil amendment by this process. This process is called “composting” and the final product of composting is called “compost”. Generally speaking there are two processes that yield compost:
- ANAEROBIC (without oxygen) decomposition.
- AEROBIC (with oxygen) decomposition and stabilization.
In these processes, bacteria, fungi, molds, protozoa, actinomycetes, and other saprophytic organisms feed upon decaying organic materials initially, while in the later stages of decomposition mites, millipedes, centipedes, springtails, beetles and earthworms further breakdown and enrich the composting materials. The organisms will vary in the pile due to temperature conditions, but the goal in composting is to create the most favorable environment possible for the desired organisms. Differences between aerobic and anaerobic composting are discussed below.
Anaerobic Decomposition (Fermentation)
Anaerobic decomposition takes place in nature, as in the decomposition of the organic muds at the bottom of marshes and in buried organic materials to which oxygen does not have access. Intensive reduction of organic matter by putrefaction is usually accompanied by disagreeable odors of hydrogen sulfide and reduced organic compounds which contain sulfur, such as mercaptans (any sulfur-containing organic compound).
Putrefactive breakdown of organic material takes place anaerobically. Organic compounds break down by the action of living organisms that do not require air in the normal sense. These organisms use nitrogen, phosphorus, and other nutrients to live and to develop cell protoplasm, but they reduce the organic nitrogen to organic acids and ammonia. The carbon from the organic compounds which is not utilized in the cell protein is liberated mainly in the reduced form of methane (CH4). A small portion of carbon may be respired as carbon dioxide (C02).
Since anaerobic destruction of organic matter is a reduction process, the final product, humus, is subject to some aerobic oxidation when put on the soil, that is, it may appear to decompose further after being exposed to air. This oxidation is minor, takes place rapidly, and is of no consequence in the utilization of the material on the soil. In other words, much less heat is generated in anaerobic decomposition than in aerobic decomposition.
The lack of heat generated in the anaerobic destruction of organic matter is a definite disadvantage if contaminated materials areused for composting. High temperatures are needed for the destruction of pathogens and parasites. In anaerobic decomposition the pathogenic organisms do eventually disappear in the organic mass, as a result of the unfavorable environment and biological antagonisms. The disappearance is slow, and the material must be held for periods of six months to a year to ensure relatively complete destruction of pathogens, such as the eggs of Ascaris, nematodes which are among the most resistant of the fecal-borne disease parasites in wastes. Therefore, make compost this year and use it next year.
However, organic material can be decomposed anaerobically to produce compost. For instance, a heavy plastic bag can be used to decompose grass clippings or other high nitrogen materials, shredded leaves, kitchen trimmings, a small amount of stable manure or other compostable materials. However, as anaerobic compost can have a strong odor (and may need to be aired prior to using), it is not usually the first choice for home owners. For more details see Structures.
Aerobic Decomposition
When organic materials decompose in the presence of oxygen, the process is called “aerobic.” The aerobic process is most common in nature. For example, it takes place on ground surfaces such as the forest floor, where droppings from trees and animals are converted into a relatively stable humus. There is no accompanying bad smell when there is adequate oxygen present.
In aerobic decomposition, living organisms, which use oxygen, feed upon the organic matter. They use the nitrogen, phosphorus, some of the carbon, and other required nutrients. Much of the carbon serves as a source of energy for the organisms and is burned up and respired as carbon dioxide (C02). Since carbon serves both as a source of energy and as an element in the cell protoplasm, much more carbon than nitrogen is needed. Generally about two-thirds of carbon is respired as C02, while the other third is combined with nitrogen in the living cells. However, if the excess of carbon over nitrogen (C:N ratio) in organic materials being decomposed is too great, biological activity diminishes. Several cycles of organisms are then required to burn most of the carbon.
When some of the organisms die, their stored nitrogen and carbon becomes available to other organisms. As other organisms use the nitrogen from the dead cells to form new cell material, once more excess carbon is converted to C02. Thus, the amount of carbon is reduced and the limited amount of nitrogen is recycled. Finally, when the ratio of available carbon to available nitrogen is in sufficient balance, nitrogen is released as ammonia. Under favorable conditions, some ammonia may oxidize to nitrate. Phosphorus, potash, and various micro-nutrients are also essential for biological growth. These are normally present in more than adequate amounts in compostable materials and present no problem.
During composting a great deal of energy is released in the form of heat in the oxidation of the carbon to C02. For example, if a gram-molecule of glucose is dissimilated under aerobic conditions, 484 to 674 kilogram calories (kcal) of heat may be released. If the organic material is in a pile or is otherwise arranged to provide some insulation, the temperature of the material during decomposition will rise to over 170°F. If the temperature exceeds 162°F to 172°F, however, the bacterial activity is decreased and stabilization is slowed down.
Initially, mesophilic organisms, which live in temperatures of 50°F to 115°F, colonize in the materials. When the temperature exceeds about 120°F, thermophilic organisms, which grow and thrive in the temperature range 115°F to 160°F., develop and replace the mesophilic bacteria in the decomposition material. Only a few groups of thermophiles carry on any activity above 160°F.
Oxidation at thermophilic temperatures takesplace more rapidly than at mesophilic temperatures and, hence, a shorter time is required for decomposition (stabilization). The high temperatures will destroy pathogenic bacteria, protozoa (microscopic one-celled animals), and weed seeds, which are detrimental to health or agriculture when the final compost is used.
Aerobic oxidation of organic matter produces no objectionable odor. If odors are noticeable, either the process is not entirely aerobic or there are some special conditions or materials present which are creating an odor. Aerobic decomposition or composting can be accomplished in pits, bins, stacks, or piles, if adequate oxygen is provided. Turning the material at intervals or other techniques for adding oxygen is useful in maintaining aerobic conditions.
Compost piles under aerobic conditions attain a temperature of 140°F to 160°F in one to five days depending upon the material and the condition of the composting operation. This temperature can also be maintained for several days before further aeration. The heat necessary to produce and maintain this temperature must come from aerobic decomposition which requires oxygen. After a period of time, the material will become anaerobic unless it is aerated.
In this manual the term “aerobic composting” will be used in its commonly accepted meaning of that process. It requires a considerable amount of oxygen and produces none of the characteristic features of anaerobic putrefaction. In its modern sense, aerobic composting can be defined as a process in which, under suitable environmental conditions, aerobic organisms, principally thermophilic, utilize considerable amounts of oxygen in decomposing organic matter to a fairly stable humus.
Requirements for Efficient Decomposition
Decomposition of organic material in the compost pile depends on maintaining microbial activity. Any factor which slows or halts microbial growth also impedes the composting process. Efficient decomposition occurs if aeration, moisture, particle size, and a sufficient source of carbon and nitrogen are in evidence.
Aeration/Oxygen
Oxygen is required for microbes to decompose organic wastes efficiently. Some decomposition occurs in the absence of oxygen (anaerobic conditions); however, the process is slow, and foul odors may develop. Because of the odor problem, composting without oxygen is not recommended in a residential setting unless the process is conducted in a fully closed system (see plastic bag method under Composting Structures). Mixing the pile once or twice a month provides the necessary oxygen and significantly hastens the composting process. A pile that is not mixed may take three to four times longer to decompose. Raising the pile off the ground allows air to be drawn through the mass as the material decomposes. Coarse materials should be placed on the bottom as the pile is built or placed in the pile and removed after the decomposition starts.
Oxygen levels should be kept at 5% throughout the entire pile. Typical oxygen percents range from 6% – 16% in the pile air spaces or in the exhausted air; and 20% at the exposed portions of the pile. Failure to keep all parts of the compost pile above the 5% oxygen level will cause the pile to “go anaerobic”, with the accompanying odor problems. The more oxygen, up to at least 10-12 percent, the more quickly the biodegradation will take place.
Moisture
Adequate moisture is essential for microbial activity. A dry compost will not decompose efficiently. Proper moisture encourages the growth of microorganisms that break down the organic matter into humus. If rainfall is limited, water the pile periodically to maintain a steady decomposition rate. Add enoughwater so the pile is damp but not soggy. Avoid over watering. Excess water can lead to anaerobic conditions which slow down the degradation process and cause foul odors. If the pile should become too wet, turn it to dry it out and restart the process.
Particle Size
Grinding the organic material before composting greatly reduces decomposition time. The smaller the size of the organic refuse particle, the more quickly it can be consumed by the microbes. A shredder is useful for chipping or shredding most landscape refuse and is essential if brush or sticks are to be composted. A low-cost method of reducing the size of fallen tree leaves is to mow the lawn before raking. Wind-rowing the leaves into long narrow piles one foot high will make the shredding process more efficient. If the mower has an appropriate bag attachment, the shredded leaves can be collected directly. However, grinding is entirely optional.
Temperature
Temperature of the compost pile is very important to the biological activity taking place. Low outside temperatures slow the activity down, while warmer temperatures speed up decomposition. The microbes that make up the bulk of the decomposition process fall into two categories: mesophilic, those that live and function in temperatures of 50 to 113°F, and thermophilic, those that thrive at temperatures between 113 to 158°F. A well-mixed, adequately working compost pile will heat to temperatures between 110°F and 160°F as the microbes actively feed on the organic materials. These high temperatures will help destroy weed seeds and disease organisms within the pile.
Organisms in Composting
There are many organisms that breakdown organic materials. Most are not seen by the human eye, but they are there throughout the process. Others that are large enough to see, are usually associated with the later breakdown stages.
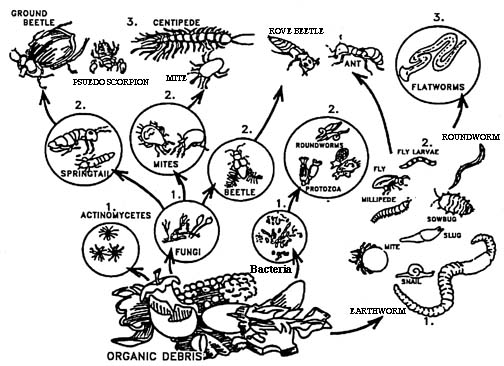
The most important organisms in the breakdown process are the bacteria. The bacteria present in any given pile are dependent upon the raw material present, amount of air in the pile, moisture conditions of the pile, pile temperature and numerous other factors. Bacteria are very small–20,000+ laid end to end may span no more that an inch. Compostable organic materials normally contain a large number and many different types of bacteria, fungi, molds, and other living organisms. Only very limited data are available regarding the variety of different organisms and their specific functions. It appears that more species of bacteria are involved in aerobic decomposition than in anaerobic putrefaction.
Although many types of organisms are required for decomposition of the different materials, the necessary variety is usually present in the materials to be composted, and the organisms thrive when environmental conditions are satisfactory. During decomposition, marked changes take place in the nature and abundance of the biological population. Some of the many species will multiply rapidly at first but will dwindle as the environment changes and other organisms are able to thrive under more varied conditions. Temperature and changes in the available food supply probably exert the greatest influence in determining the species of organisms comprising the population at any one time. Aerobic composting is a dynamic process in which the work is done by the combined activities of a wide succession of mixed bacteria, actinomycetes, fungi, and other biological populations. Since each is suited to a particular environment of relatively limited duration and each is most active in decomposition of some particular type of organic matter, the activities of one group complement those of another. The mixed populations parallel the complex environments afforded by the heterogeneous nature of the compostable material. Except for short periods during turning, the temperature increases steadily in proportion to the amount of biological activity until equilibrium (state of balance) with subsequent heat losses is reached, or the material becomes well-stabilized (humus-like).
In aerobic composting bacteria, actinomycetes, and fungi are the most active. Mesophilic (low temperature 50-115°F) bacteria are characteristically predominant at the start and in the early part of the process, soon giving way to thermophilic (high temperature 110-150+°F) bacteria which inhabit all parts of the stack where the temperature is satisfactory, eventually most of the stack. Thermophilic fungi usually appear after 5 to 10 days, and actinomycetes become prominent in the final stages, when short duration, rapid composting is accomplished. Except in the final stages of the composting period, when the temperature drops, actinomycetes and fungi are confined to a sharply defined outer zone of the stack, 2 to 6 inches in thickness, beginning just under the outer surface. Some molds also grow in this outer zone. The population of fungi and actinomycetes is often great enough to impart a distinctly grayish white appearance to this outer zone.
The sharply defined inner and outer limits of the shell (in which actinomycetes and fungi grow during the high temperature active composting period) are due to the inability of these organisms to grow at the higher temperatures of the interior of the pile. The thermophilic actinomycetes and fungi have been found to grow at temperatures between 120° and 150°F. Frequent turning–such as is sometimes necessary for fly control–inhibits their growth, since the cooler outer shell is turned into the interior before they can develop in large numbers.
Various investigations have shown that many different types of thermophilic bacteria apparently play a major part in decomposing protein and other organic matter. They appear to be solely responsible for the intense activity characteristic of the first few days, when the temperature reaches 150° to 170°F and major changes in the nature of the compost pile are taking place, i.e., when the pile is drastically shrinking and the appearance of the material is undergoing rapid change. They continue to predominate throughout the process in theinterior of the piles, where temperatures are inhibitory to actinomycetes and fungi.
In spite of being confined primarily to the outer layers and becoming active only during the latter part of the composting period, fungi and actinomycetes play an important role in the decomposition of cellulose, lignins, and other more resistant materials, which are attacked after the more readily decomposed materials have been utilized. There are many bacteria which attack cellulose. However, in the parts of compost piles populated chiefly by bacteria, cellulose (paper) breaks down very little, whereas in the layers or areas inhabited by actinomycetes and fungi it becomes almost unrecognizable. Considerable cellulose and lignin decomposition by actinomycetes and fungi can occur near the end of the composting period when the temperatures have begun to drop and the environment in a larger part of the pile is satisfactory for their growth.
It should be noted that since the necessary organisms for composting are usually present and will carry on the process when the environment is suitable, an extensive knowledge of the characteristics of the various organisms is not necessary for understanding a compost pile. Normal maintenance as described in this manual will help to insure proper balance and numbers of beneficial microorganisms.
Since decomposition is the crux of the composting process, let’s take a look at the various organisms that play an essential role in the working compost heap. Most are microscopic, some are large enough to be observed with the unaided eye, but all are beneficial, each having a role in breaking down raw organic matter into finished compost. They are known as decomposers. By far the most important microscopic decomposers are bacteria, which do the lion’s share of decomposition in the compost heap. But there are other microscopic creatures such as actinomycetes, fungi, and protozoa, that also play an important role. Together, these are chemical decomposers that change the chemistry of the organic wastes. The largerfauna in the heap include mites, millipedes, flatworms, centipedes, sowbugs, snails, slugs, spiders, springtails, beetles, ants, flies, nematodes and, most importantly, earthworms. Collectively, these are called the physical decomposers since they bite, grind, suck, tear and chew the materials into smaller pieces, making them more suitable for the chemical work of the microscopic decomposers.
All of the organisms, from the microscopic bacteria to the largest of the physical decomposers, are part of a complex food chain in the compost pile. They can be categorized as first, second and third level consumers, depending upon whom they eat and by whom they are eaten. First level consumers attract and become the food of second level consumers, who in turn are consumed by third level consumers. The organisms comprising each level of the food chain serve to keep the populations of the next lower level in check, so that a balance can be maintained throughout the compost. For example, according to Daniel L. Dindal, in Ecology of Compost:
. . . mites and springtails eat fungi. Tiny feather-winged beetles feed on fungal spores. Nematodes ingest bacteria. Protozoa and rotifers present in water films feed on bacteria and plant particles. Predaceous mites and pseudo- scorpions prey upon nematodes, fly larvae, other mites and collembolans. Free-living flatworms ingest gastropods, earthworms, nematodes and rotifers. Third level consumers such as centipedes, rove beetles, ground beetles, and ants prey on second level consumers. These creatures function best at medium or mesophilic temperatures, so they will not be in the pile at all times.
Chemical Decomposers
These organisms are the initial inhabitants of the pile. Many of them are unseen and come in with the materials that make up the pile. These organisms are around all of the time and only need to find the conditions right “to their liking” in order to start their normal functions of breaking down organic materials.
Bacteria
Bacteria likely to be found in a compost heap are aerobic bacteria that specialize in breaking down organic compounds and thrive in temperatures ranging up to 170°F (77°C). Bacterial populations differ from pile to pile, depending upon the raw materials of the compost, degree of heat, amount of air present, moisture level, geographical location of the pile, and other considerations. Bacteria are single-celled and can be shaped like a sphere, rod, or a spiral twist. They are so small that it would take 25,000 bacteria laid end to end to take up one inch on a ruler, and an amount of garden soil the size of a pea may contain up to a billion bacteria. Most bacteria are colorless and cannot make carbohydrates from sunshine, water, and carbon dioxide the way more complex green plants can. Some bacteria produce colonies; others are free-living. All reproduce by means of binary fission.
In binary fission, the nucleus splits in two and a new cell wall grows crosswise over the middle of the cell. Each half contains one of the two nuclei, so that a new individual is produced from a single bacterial cell.
Under the best conditions, a colony of bacteria can multiply into billions in a very short time. The life span of one generation of bacteria is about 20 to 30 minutes, so that one cell may yield a progeny of billions of individuals in half a day.
Bacteria are the most nutritionally diverse of all organisms, which is to say, as a group, they can eat nearly anything. Most compost bacteria are heterotrophic, meaning that they can use living or dead organic materials. Some are so adaptable that they can use more than a hundred different organic compounds as their source of carbon because of their ability to produce a variety of enzymes. Usually, they can produce the appropriate enzyme to digest whatever material they find themselves on. In addition, respiratory enzymes in the cell membrane make aerobic respiration possible as an energy source for compost bacteria.
Since bacteria are smaller, less mobile and less complex than most organisms, they are less able to escape an environment that becomes unfavorable. A decrease in the temperature of the pile or a sharp change in its acidity can render bacteria inactive or kill them. When the environment of a heap begins to change, bacteria that formerly dominated may be decimated by another species.
Actinomycetes
The characteristically earthy smell of newly plowed soil in the spring is caused by actinomycetes, a higher form of bacteria similar to fungi and molds. Actinomycetes are especially important in the formation of humus. While most bacteria are found in the top foot or so of topsoil, actinomycetes may work many feet below the surface. Deep under the roots they convert dead plant matter to a peat-like substance. While they are decomposing animal and vegetable matter, actinomycetes liberate carbon, nitrogen and ammonia, making nutrients available for higher plants. They are found on every natural substrate, and the majority are aerobic and mesophilic. Five percent or more of the soil’s bacterial population is comprised of actinomycetes.
The reason bacteria tend to die rapidly as actinomycete populations grow in the compost pile is that actinomycetes have the ability to produce antibiotics, chemical substances that inhibit bacterial growth.
Protozoa
Protozoa are the simplest form of animal organism. Even though they are single-celled and microscopic in size, they are larger and more complex in their activities than most bacteria. A gram of soil can contain as many as a million protozoa, but a gram of compost has many thousands less, especially during the thermophilic stage. Protozoa obtain their food from organic matter in the same way bacteriado, but because they are present in far fewer numbers than are bacteria, they play a much smaller part in the composting process.
Fungi
Fungi are many-celled, filamentous or single-celled primitive plants. Unlike more complex green plants, they lack chlorophyll, and, therefore, lack the ability to make their own carbohydrates. Most of them are classified as saprophytes because they live on dead or dying material and obtain energy by breaking down organic matter in dead plants and animals. Like the actinomycetes, fungi take over during the final stages of the pile when the compost has been changed to a more easily digested form. The best temperature for active fungi in the compost heap is around 70° to 75°F though some thermophilic forms prefer much greater heat and survive to 120°F.
Physical Decomposers
The larger organisms that chew and grind their way through the compost heap are higher up in the food chain and are known as physical decomposers. The following is a rundown of some of the larger physical decomposers that you may find in nearly any compost heap. Most of these creatures function best at medium or mesophilic temperatures, so they will not be in the pile at all times.
Mites
Mites are related to ticks, spiders, and horseshoe crabs because they have in common six leg-like, jointed appendages. They can be free-living or parasitic, sometimes both at once. Some mites are small enough to be invisible to the naked eye, while some tropical species are up to a half-inch in length. Mites reproduce very rapidly, moving through larval, nymph, adult and dormant stages. They attack plant matter, but some are also second level consumers, ingesting nematodes, fly larvae, other mites and springtails.
Millipedes
The wormlike body of the millipede has many leg-bearing segments, each exceptthe front few bearing two pairs of walking legs. The life cycles are not well understood, except that eggs are laid in the soil in springtime, hatching into small worms. Young millipedes molt several times before gaining their full complement of legs. When they reach maturity, adult millipedes can grow to a length of 1 to 2 inches. They help break down plant material by feeding directly on it.
Centipedes
Centipedes are flattened, segmented worms with 15 or more pairs of legs, 1 pair per segment. They hatch from eggs laid during the warm months and gradually grow to their adult size. Centipedes are third level consumers, feeding only on living animals, especially insects and spiders.
Sowbugs
The sowbug is a fat-bodied, flat creature with distinct segments. In structure, it resembles the crayfish to which it is related. Sowbugs reproduce by means of eggs that hatch into smaller versions of the adults. Since females are able to deposit a number of eggs at one time, sowbugs may become abundant in a compost heap. They are first level consumers, eating decaying vegetation.
Snails and Slugs
Both snails and slugs are mollusks and have muscular disks on their undersides that are adapted for a creeping movement. Snails have a spirally curved shell, a broad retractable foot, and a distinct head. Slugs, on the other hand, are so undifferentiated in appearance that one species is frequently mistaken for half of a potato. Both snails and slugs lay eggs in capsules or gelatinous masses and progress through larval stages to adulthood. Their food is generally living plant material, but they will attack fresh garbage and plant debris and will appear in the compost pile. It is well,therefore, to look for them when you spread your compost, for if they move into your garden, they can do damage to crops.
Spiders
Spiders, which are related to mites, are one of the least appreciated animals in the garden. These eight-legged creatures are third level consumers that feed on insects and small invertebrates, and they can help control garden pests.
Springtails
Springtails are very small insects, rarely exceeding one-quarter inch in length. They vary in color from white to blue-grey or metallic and are mostly distinguished by their ability to jump when disturbed. They feed by chewing decomposing plants, pollen, grains, and fungi.
Beetles
The rove beetle, ground beetle, and feather-winged beetle are the most common beetles in compost. Feather-winged beetles feed on fungal spores, while the larger rove and ground beetles prey on other insects as third level consumers. Beetles are easily visible insects with two pairs of wings, the more forward-placed of these serving as a cover or shield for the folded and thinner back-set ones that are used for flying. A beetle’s immature stage is as a soft-skinned grub that feeds and grows during the warm months. Once grubs are full grown, they pass through a resting or pupal stage and change into hard-bodied, winged adults. Most adult beetles, like the larval grubs of their species, feed on decaying vegetables, while some, like the rove and ground beetles, prey on snails, insects, and other small animals. The black rove beetle is an acknowledged predator of snails and slugs. Some people import them to their gardens when slugs become a garden problem.
Ants
Ants feed on a variety of material, including aphid honeydew, fungi, seeds, sweets, scraps, other insects, and sometimes other ants. Compost provides some of these foods, and it also provides shelter for nests and hills. They will remain, however, only while the pile is relatively cool. Ants prey on first level consumers, and may benefit the composting process by bringing fungi and other organisms into their nests. The work of ants can make compost richer in phosphorus and potassium by moving minerals from one place to another.
Flies
Many flies, including black fungus gnats, soldier flies, minute flies, and houseflies, spend their larval phase in compost as maggots. Adults can feed upon almost any kind of organic material. All flies undergo egg, larval, pupal, and adult stages. The eggs are laid in various forms of organic matter. Houseflies are such effective distributors of bacteria that when an individual fly crawls across a sterile plate of lab gelatin, colonies of bacteria later appear in its tracks. You can see how during the early phases of the composting process, flies provide ideal airborne transportation for bacteria on their way to the pile. If you keep a layer of dry leaves or grass clippings on top of your pile and cover your garbage promptly while building compost, your pile will not provide a breeding place for horseflies, mosquitoes, or houseflies which may become a nuisance to humans. Fly larvae will not survive the thermophilic temperatures in the well-managed compost pile. Mites and other organisms in the pile also keep fly larvae reduced in number. However, though many flies die with the coming of frost, the rate of reproduction is so rapid that a few survivors can repopulate an area before the warm season has progressed very far.
Worms
Nematodes or eelworms, free-living flatworms, and rotifers all can be found in compost. Nematodes are microscopic creatures that can be classified into three categories: those that live on decaying organic matter; those that arepredators on other nematodes, bacteria, algae, protozoa, etc.; and those that can be serious pests in gardens where they attack the roots of plants. Flatworms, as their name implies, are flattened organisms that are usually quite small in their free-living form. Most flatworms are carnivorous and live in films of water within the compost structure. Rotifers are small, multicellular animals that live freely or in tubes attached to a substrate in the pile. Their bodies are round and divisible into three parts, a head, trunk, and tail. They are generally found in films of water and many forms are aquatic. The rotifers in compost are found in water which adheres to plant substances where they feed on microorganisms.
Earthworms
If bacteria are the champion microscopic decomposers, then the heavyweight champion is doubtlessly the earthworm. Pages of praise have been written to the earthworm, ever since it became known that this creature spends most of its time tilling and enriching the soil. The great English naturalist, Charles Darwin, was the first to suggest that all the fertile areas of this planet have at least once passed through the bodies of earthworms.
The earthworm consists mainly of an alimentary canal which ingests, decomposes, and deposits casts continually during the earthworm’s active periods. As soil or organic matter is passed through an earthworm’s digestive system, it is broken up and neutralized by secretions of calcium carbonate from calciferous glands near the worm’s gizzard. Once in the gizzard, material is finely ground prior to digestion. Digestive intestinal juices rich in hormones, enzymes, and other fermenting substances continue the breakdown process. The matter passes out of the worm’s body in the form of casts, which are the richest and finest quality of all humus material. Fresh casts are markedly higher in bacteria, organic material, available nitrogen, calcium and magnesium, and available phosphorus and potassium than soil itself.
Earthworms thrive on compost and contributeto its quality through both physical and chemical processes, and reproduce readily in the well-managed pile. Since earthworms are willing and able to take on such a large part in compost making, it is the wise gardener who adjust his composting methods to take full advantage of the earthworm’s special talents.
Use of Inocula
Composting developments have been accompanied by considerable discussion of the importance of special inocula (bacterial activators), supposedly containing several pure strains of laboratory organisms or other biological factors essential in the decomposition of organic matter and nitrogen fixation, e.g., “enzymes,” “hormones,” “preserved living organisms,” “activated factors,” “biocatalyst,” etc. In fact, several commercial composting processes are built around the use of some special inoculum, often known only to its discoverer and proponent, who claims it to be fundamental to the successful operation of the process. The need of such inocula has always been debatable, and most composting studies have strongly indicated that they are unnecessary.
That inocula in composting organic waste containing refuse, manure, vegetable wastes, etc. are not necessary or advantageous seems logical, since bacteria are always present in very large numbers in such material and can be eliminated only by drastic sterilization methods. In any case, the number of bacteria is rarely a limiting factor in composting. Provided that the environmental factors are appropriate, the indigenous bacteria, which are much better adapted than forms produced under laboratory conditions, multiply rapidly. Thus the rate of composting is governed simply by the environmental conditions.
Special enzyme preparations are also advertised as necessary compost “starters.” However, the vast number of enzymes involved in decomposition, as well as the difficulty and expense involved in isolating and synthesizing them, would make initiating the composting process with enzymes alone highly impracticaleven if satisfactory preparations were available. The addition of enzymes to raw compostable materials is unnecessary because bacteria synthesize efficiently and rapidly all the enzymes required.
Another popular starter is “hormones,” a term popularly used to designate the growth factors and vitamins needed by bacteria or other organisms. The organic constituents of mixed compostable materials usually contain all the growth factors needed for normal growth. Also, growth factors and vitamins are produced by micro-organisms and will undoubtedly be produced in sufficient quantities in a mixed microbial population to meet normal requirements.
A third group of additives are “biocatalyst” or “activated factors,” various biological materials which are supposed to activate and accelerate decomposition and stabilization of organic material.
In some cases the “activator” supplies some material which is lacking in the compost. For example, straw or paper, which does not contain the necessary biological nutrients, is not readily composted alone, but if nitrogen and phosphorus are added, the straw and paper will serve as the carbon source for decomposition.
Agricultural experimentation has found starters or additives usually unnecessary. In one experiment, horse manure, compost material, normal soil, and special commercially prepared bacterial cultures were mixed with garbage and refuse to form one set of compost piles. In another set of piles, materials were composted without these special commercially prepared bacterial cultures. It was found that, although rich in bacteria, none of the inocula accelerated the composting process or improved the final product. There was no significant difference in the temperature curves or in the chemical analyses of the material at different intervals during the composting period. Therefore, it was concluded that when the environment is appropriate, the varied indigenous biological population will multiply rapidly and composting is not delayed.
The information given herein is for educational purposes only. Reference to commercial products or trade names is made with the understanding that no discrimination is intended and no endorsement by the Texas AgriLife Extension Service is implied.
Educational programs of the Texas AgriLife Extension Service are open to all people without regard to race, color, sex, disability, religion, age, or national origin.
Publication Revised February 2009